Vascular reactivity in old spontaneously hypertensive stroke-prone rats- effects of in vitro ischemia/reperfusion injury
Article Information
Sevil Korkmaz-Icöz1*, Mona Isabella Benker1, Shiliang Li1, Sivakkanan Loganathan1,2, Patricia Kraft1, Tobias Mayer1, Sandra Schubach1, Paige Brlecic1, Alex Ali Sayour1,3, Tamás Radovits3, Matthias Karck1, Gábor Szabó1,2
1Department of Cardiac Surgery, University Hospital Heidelberg, 69120 Heidelberg, Germany
2Department of Cardiac Surgery, University Hospital Halle (Saale), 06120 Halle, Germany
3Heart and Vascular Center, Semmelweis University, 1122 Budapest, Hungary
*Corresponding author: Sevil Korkmaz-Icöz, Department of Cardiac Surgery, University Hospital Heidelberg, 69120 Heidelberg, Germany
Received: 07 June 2021; Accepted: 15 June 2021; Published: 04 August 2021
Citation:
Sevil Korkmaz-Icöz, Mona Isabella Benker, Shiliang Li, Sivakkanan Loganathan, Patricia Kraft, Tobias Mayer, Sandra Schubach, Paige Brlecic, Alex Ali Sayour, Tamás Radovits, Matthias Karck, Gábor Szabó. Vascular reactivity in old spontaneously hypertensive stroke-prone rats- effects of in vitro ischemia/reperfusion injury. Journal of Surgery and Research 4 (2021): 385-398.
View / Download Pdf Share at FacebookAbstract
Objectives: Peripheral arterial disease affects mainly the aorta and lower extremities and commonly results in tissue ischemia. Medical interventions to restore blood flow can induce reperfusion injury. We investigated the effects of both hypertension and ageing on vascular graft responsiveness under normoxia and ischemia/reperfusion (IR) conditions.
Methods: Hemodynamic changes and aortic morphometry were studied in 18-month-old spontaneously hypertensive stroke prone (SHRSP, n=8) and age-matched normotensive Wistar (control, n=8) rats. Whereas thoracic aortic rings of the control- and SHRSP groups were immediately mounted in organ bath chambers (normoxia-group), aortic segments in the control-IR and SHRSP-IR groups underwent cold ischemic preservation, prior to mounting.
Results: Significantly increased intima-media thickness, intima-media cross-sectional area, and lumen normalized to body weight were observed in SHRSP displaying a systolic blood pressure of 205±16 mmHg compared to controls 124±8 mmHg, p < 0.05. Phenylephrine-induced vasoconstriction was greater in aorta of SHRSP compared to controls. IR injury increased contractile responses to phenylephrine in both control and SHRSP compared to the corresponding groups, which was further increased in SHRSP-IR compared to control-IR (as percentage of KCl-contraction: SHRSP-IR 167±26% vs. control-IR 101±5%, p < 0.05). Compared to controls, vasoconstrictive response to high K+-induced depolarization was significantly reduced in SHRSP, control-IR, and SHRSP-IR. Additionally, KCl-induced contraction was reduced in SHRSP-IR compared to control-IR (SHRSP-IR 0.7±0.1g vs. control-IR 2.5±0.1g, p < 0.05). IR reduced maximum endothelium-dependent relaxation to acetylcholine in the normoxia contr
Keywords
Aging, Hypertension, Aorta, Vascular tone, Spontaneously hypertensive stroke-prone rats
Aging articles; Hypertension articles, Aorta articles; Vascular tone articles; Spontaneously hypertensive stroke-prone rats articles
Aging articles Aging Research articles Aging review articles Aging PubMed articles Aging PubMed Central articles Aging 2023 articles Aging 2024 articles Aging Scopus articles Aging impact factor journals Aging Scopus journals Aging PubMed journals Aging medical journals Aging free journals Aging best journals Aging top journals Aging free medical journals Aging famous journals Aging Google Scholar indexed journals Hypertension articles Hypertension Research articles Hypertension review articles Hypertension PubMed articles Hypertension PubMed Central articles Hypertension 2023 articles Hypertension 2024 articles Hypertension Scopus articles Hypertension impact factor journals Hypertension Scopus journals Hypertension PubMed journals Hypertension medical journals Hypertension free journals Hypertension best journals Hypertension top journals Hypertension free medical journals Hypertension famous journals Hypertension Google Scholar indexed journals Aorta articles Aorta Research articles Aorta review articles Aorta PubMed articles Aorta PubMed Central articles Aorta 2023 articles Aorta 2024 articles Aorta Scopus articles Aorta impact factor journals Aorta Scopus journals Aorta PubMed journals Aorta medical journals Aorta free journals Aorta best journals Aorta top journals Aorta free medical journals Aorta famous journals Aorta Google Scholar indexed journals Vascular tone articles Vascular tone Research articles Vascular tone review articles Vascular tone PubMed articles Vascular tone PubMed Central articles Vascular tone 2023 articles Vascular tone 2024 articles Vascular tone Scopus articles Vascular tone impact factor journals Vascular tone Scopus journals Vascular tone PubMed journals Vascular tone medical journals Vascular tone free journals Vascular tone best journals Vascular tone top journals Vascular tone free medical journals Vascular tone famous journals Vascular tone Google Scholar indexed journals Spontaneously hypertensive stroke-prone rats articles Spontaneously hypertensive stroke-prone rats Research articles Spontaneously hypertensive stroke-prone rats review articles Spontaneously hypertensive stroke-prone rats PubMed articles Spontaneously hypertensive stroke-prone rats PubMed Central articles Spontaneously hypertensive stroke-prone rats 2023 articles Spontaneously hypertensive stroke-prone rats 2024 articles Spontaneously hypertensive stroke-prone rats Scopus articles Spontaneously hypertensive stroke-prone rats impact factor journals Spontaneously hypertensive stroke-prone rats Scopus journals Spontaneously hypertensive stroke-prone rats PubMed journals Spontaneously hypertensive stroke-prone rats medical journals Spontaneously hypertensive stroke-prone rats free journals Spontaneously hypertensive stroke-prone rats best journals Spontaneously hypertensive stroke-prone rats top journals Spontaneously hypertensive stroke-prone rats free medical journals Spontaneously hypertensive stroke-prone rats famous journals Spontaneously hypertensive stroke-prone rats Google Scholar indexed journals Peripheral arterial disease articles Peripheral arterial disease Research articles Peripheral arterial disease review articles Peripheral arterial disease PubMed articles Peripheral arterial disease PubMed Central articles Peripheral arterial disease 2023 articles Peripheral arterial disease 2024 articles Peripheral arterial disease Scopus articles Peripheral arterial disease impact factor journals Peripheral arterial disease Scopus journals Peripheral arterial disease PubMed journals Peripheral arterial disease medical journals Peripheral arterial disease free journals Peripheral arterial disease best journals Peripheral arterial disease top journals Peripheral arterial disease free medical journals Peripheral arterial disease famous journals Peripheral arterial disease Google Scholar indexed journals hypertensive vasculopathy articles hypertensive vasculopathy Research articles hypertensive vasculopathy review articles hypertensive vasculopathy PubMed articles hypertensive vasculopathy PubMed Central articles hypertensive vasculopathy 2023 articles hypertensive vasculopathy 2024 articles hypertensive vasculopathy Scopus articles hypertensive vasculopathy impact factor journals hypertensive vasculopathy Scopus journals hypertensive vasculopathy PubMed journals hypertensive vasculopathy medical journals hypertensive vasculopathy free journals hypertensive vasculopathy best journals hypertensive vasculopathy top journals hypertensive vasculopathy free medical journals hypertensive vasculopathy famous journals hypertensive vasculopathy Google Scholar indexed journals vascular damage articles vascular damage Research articles vascular damage review articles vascular damage PubMed articles vascular damage PubMed Central articles vascular damage 2023 articles vascular damage 2024 articles vascular damage Scopus articles vascular damage impact factor journals vascular damage Scopus journals vascular damage PubMed journals vascular damage medical journals vascular damage free journals vascular damage best journals vascular damage top journals vascular damage free medical journals vascular damage famous journals vascular damage Google Scholar indexed journals
Article Details
1. Introduction
Peripheral arterial disease (PAD) is an arterial occlusive disease affecting mainly the aorta and lower extremities, resulting in ischemia. Hypoxic insult impairs the regulation of vascular smooth muscle (VSM) tone by altering the contractile properties of the vascular wall. Paradoxically, the reperfusion process itself can cause further damage on top of ischemia. During reperfusion, polymorphonuclear leucocytes accumulate in the ischemic tissue, and reactive oxygen species are generated, resulting in impaired vascular function and altered tissue barrier functions [1]. Hypertension is defined as repeatedly elevated systolic (≥ 140 mmHg) and/or diastolic blood pressures (≥ 90 mmHg) [2]. Essential hypertension, also known as primary or idiopathic hypertension, constitutes 80-95% of cases and the precise cause is poorly understood. Secondary hypertension, accounting for 5-15% of all patients, is due to a specific disease or abnormality. If left untreated, the sustained increase in blood pressure can lead to structural damage of the heart, coronary vascular and endothelial changes, and alterations in the cardiac conduction system. Hypertension acts as a common risk factor for PAD. It is well-known that the vascular wall is a target organ in hypertension-related damage. From a hemodynamic perspective, one of the most important factors underlying the development of hypertension is increased vascular sensitivity to vasoconstrictive stimuli. This is largely due to alterations in arterial structure and function, leading to an increase in total peripheral vascular resistance [3]. It is well established that ageing is associated with changes in the morphological and functional characteristics of VSM and we have reported alterations in endothelium-dependent relaxation and vasocontractile responses [4-6]. Additionally, persistently elevated blood pressure can lead to vascular structural changes (remodelling), hyperplasia of VSM, and increasing arterial stiffening. Thus, pre-existent vascular damage, due to age and hypertension, may be aggravated by reperfusion therapy in patients with occlusive PAD and older hypertensive patients may run a high risk for vascular complications. The spontaneously hypertensive stroke-prone rat (SHRSP) is a genetic model of severe hypertension and cerebral stroke [7,8]. The observed vascular dysfunction is similar to the pathophysiology of PAD. Some features of hypertension in this model are VSM remodelling (growth rate increase), impaired endothelium-dependent relaxation necrosis or sclerosis, and cardiac hypertrophy [9-11]. The SHRSP thus offers a convenient model for studying the pathogenesis of hypertensive vasculopathy. Although preclinical and clinical studies have examined changes induced by hypertension in the vasculature, data describing vascular damage induced by both ageing and high blood pressure after IR injury is limited. In the present study, we therefore investigated the influence of IR on changes in thoracic aortic rings, as a model of a conductance vessel, obtained from spontaneously hypertensive and normotensive control in 18-month-old rats.
2. Methods
2.1 Animals
Male SHRSP (n=8) and age-matched Wistar control rats (n=8) (Charles River, Sulzfeld, Germany) were studied at the age of 18 months. The animals were housed at a constant room temperature (22±2°C) and 12h-light/dark cycles, with free access to standard laboratory rat diet and water. The study conforms with the Guide for the Care and Use of Laboratory Animals published by the US National Institutes of Health (NIH Publication No. 85-23, revised 1996). All procedures with, and handling of, the animals during the investigations were reviewed and approved by the appropriate institutional review committee.
2.2 Measurement of arterial blood pressure and heart rate
Rats were anesthetized with sodium pentobarbital (60 mg/kg, i.p.), placed on controlled heating pads maintaining their core temperature at 37°C, tracheotomised, intubated, and artificially ventilated. A 2F-microtip pressure-volume catheter (SPR-838, Millar Instruments, Houston, TX, USA) was inserted into the right carotid artery and advanced into the ascending aorta. The systolic (SBP) and diastolic (DBP) blood pressures, and heart rate (HR) were measured, the mean arterial pressure (MAP) was calculated.
2.3 Rat model of endothelial dysfunction induced by cold ischemic storage and reperfusion
The protocol has been previously described in detail [12-14].
2.3.1 Preparation of aortic rings: After hemodynamic measurements, the descending thoracic aorta was carefully removed and quickly transferred to cold (+4°C) Krebs-Henseleit-solution (118 mM NaCl, 4.7 mM KCl, 1.2 mM KH2PO4, 1.2 mM MgSO4, 1.77 mM CaCl2, 25 mM NaHCO3, 11.4 mM glucose; pH=7.4) or cold physiological saline solution. The aorta was cleaned of periadventitial fat and surrounding connective tissue, and it was cut transversely into 4-mm width rings.
2.3.2 Conservation of aortic rings and experimental groups: Aortic rings in the control-IR (n=31 rings, 8 rats) and SHRSP-IR (n=28 rings, 8 rats) groups were stored for 24h at 4°C in closed, air-free 5 ml tubes filled with physiological saline. The tubes were previously equilibrated with nitrogen to extrude oxygen from the solution. After cold ischemic conservation, the rings proceeded to the organ bath. To stimulate free radical burst, which usually occurs during reperfusion in vivo, 200 µM hypochlorite were added to the baths for 30 min. Aortic rings in the control (n=26 rings, 8 rats) and SHRSP (n=23 rings, 8 rats) normoxia-groups did not undergo cold ischemic storage, and hypochlorite incubation, but were immediately mounted in organ baths.
2.3.3 Ex vivo organ bath experiments: As previously reported, the aortic rings were mounted on stainless steel hooks under 2g of resting tension in individual organ baths (Radnoti Glass Technology, Monrovia, CA), containing 30 ml of Krebs-Henseleit-solution, continuously gassed with 95% O2-5% CO2 and warmed to 37°C [15-17]. The tissue was then equilibrated for 60 min. During this period, the tension was periodically adjusted to the desired level, and the Krebs-Henseleit-solution was changed every 30min as a precaution against interfering metabolites. Potassium chloride (KCl) was used to test viability, prepare the vessel rings for stable contractions and reproducible dose-response curves to other vasoactive agents. At the beginning of each experiment, maximal contraction forces to KCl (80 mM) were determined and aortic rings were washed until resting tension was again obtained. An α-adrenergic receptor agonist, phenylephrine (PE, 10-9 – 10-5 M) was used to precontract the rings until a stable plateau was reached, and the relaxation responses were examined by adding cumulative concentrations of endothelium-dependent vasorelaxant acetylcholine (ACh, 10-9 - 10-4 M) and endothelium-independent dilator sodium nitroprusside (SNP, 10-10 - 10-5 M). The contractility of PE was evaluated as a percentage of KCl response and the relaxation is expressed as percent of contraction induced by PE. The half-maximal effective concentration (EC50) was determined from each individual concentration-response curve to PE, ACh, or SNP by sigmoidal fits using Origin 7.0 (Microcal Software, Northampton, Massachusetts, USA). The sensitivity pD2 (-logEC50) was then calculated.
2.3 Histology
Five-µm thick aortic sections were stained with hematoxylin and eosin as described elsewhere [17]. The wall thickness, wall and lumen cross-sectional areas were determined under a microscope using Cell^A software (Olympus Soft Imaging Solutions GmbH, Germany), wall/lumen ratio was calculated, and normalized to body weight [18]. The evaluation from four randomized non-overlapping fields of the aorta was done in a blinded fashion.
2.4 Western Blotting
The protein expression of endothelial nitric oxide synthase (eNOS) (1:1000, Abcam Cambridge, UK), hypoxia inducible factor (HIF)-1α, and heme-oxygenase (HO)-1 (1:2000, Abcam, Cambridge, UK) in aortas was performed by Western blotting. Glyceraldehyde-3-phosphate dehydrogenase (GAPDH), housekeeping proteins was used as loading control and for protein normalization.
2.5 Chemical reagents
PE, ACh, SNP and KCl were purchased from Sigma-Aldrich, Steinheim, Germany and dissolved in 0.9% NaCl. Sodium pentobarbital was purchased from Merial GmbH (Halbergmoos, Germany).
2.6 Statistical analysis
All data is expressed as mean ± standard error of the mean (SEM). Statistical analyses were performed using GraphPad Prism 7.02 software (GraphPad Software, Inc., CA, USA). In cases of hemodynamic and morphometric measurements, the Shapiro-Wilk normality test was used to assess deviations from normal distribution before statistical tests were applied. For data with normal distribution, two-sample Student’s t-test was used to analyze the differences between the control and SHRSP groups. For quantitative analysis of vascular function (Table 3) and western blot results the Shapiro-Wilk normality test was used to assess normality of data distribution. For data with normal distribution, one-way ANOVA followed by Tukey’s post-hoc test was carried out for multiple comparisons. If the data were not normally distributed, the nonparametric Kruskal–Wallis test followed by Dunn’s post-hoc test was used. In cases of cumulative concentration-response curves to PE, ACh and SNP, a two-factor mixed ANOVA (factors: hypertension and concentration of reagents [PE, ACh, SNP]) and followed by Tukey’s post-hoc test was used for multiple comparisons. A value of two-tailed p < 0.05 was considered statistically significant.
3. Results
3.1 Effects of high blood pressure in aorta of aged rats
3.1.1 General characteristics: Recordings of arterial blood pressure in the ascending aorta by a pressure catheter showed that while the SBP, DBP, and MAP were significantly higher, the HR remained unchanged in SHRSP rats as compared to the control group (Table 1).
Control |
SHRSP |
|
Systolic blood pressure (mmHg) |
124 ± 8 |
205 ± 16* |
Diastolic blood pressure (mmHg) |
101 ± 7 |
161 ± 10* |
Mean arterial pressure (mmHg) |
109 ± 7 |
176 ± 12* |
Table 1: Hemodynamic parameters
Data is represented as mean ± SEM. n=6-8 rats in each group. * p < 0.05 vs. Control. SHRSP indicates spontaneously hypertensive stroke-prone rats.
3.1.2 Aortic morphometry: SHRSP rats showed significantly lower body weight compared to the control group (326 ± 5g vs 673 ± 21g, p < 0.001). Morphometrical analyses of aortas revealed that wall thickness, wall cross-sectional area, the lumenal area normalized to body weight, and the wall:lumen area ratio were significantly higher in SHRSP rats compared to controls group (Table 2, Figure 1).
Control |
SHRSP |
|
Lumen area (mm2) |
2.20 ± 0.04 |
1.81 ± 0.08* |
Lumen area to body weight (mm2/g) (x1,000) |
3.29 ± 0.10 |
5.56 ± 0.24* |
Wall area (mm2) |
0.857 ± 0.015 |
0.864 ± 0.051 |
Wall area to body weight (mm2/g) (x1,000) |
1.28 ± 0.04 |
2.66 ± 0.18* |
Wall/lumen area ratio |
0.390 ± 0.006 |
0.478 ± 0.022* |
Wall thickness (µm) |
140.9 ± 3.3 |
151.1 ± 4.8 |
Wall thickness to body weight (µm/g) (x1,000) |
0.21 ± 0.01 |
0.46 ± 0.02* |
Table 2: Intima-media thickness, intima-media area, and lumen wall area
Data is represented as mean ± SEM. n=8 rats. * p < 0.05 vs. Control. SHRSP indicates spontaneously hypertensive stroke-prone rats.

Figure 1: Aortic morphometry. Representative hematoxylin and eosin stained sections of thoracic aortas from control and SHRSP rats used to measure intima-media wall and lumen area (top panels, x4 magnification, bar = 500 µm) and the intima-media wall thickness (bottom panels, x10 magnification, bar = 200 µm). Data is represented as mean ± SEM. n=8 rats/group. * p < 0.05 vs. Control. SHRSP indicates stroke-prone spontaneously hypertensive rats.
3.2 Effects of in vitro IR injury in aorta of aged hypertensive rats
3.2.1 Contractile responses of aortic rings: PE-induced vasoconstriction was greater in aorta of SHRSP rats compared to controls. IR injury significantly increased contractile responses to PE in both control and SHRSP rats compared to the corresponding groups, which was further increased in SHRSP-IR rings compared to control-IR group (Table 3, Figure 2A).
Control |
SHRSP |
Control-IR |
SHRSP-IR |
|
KCl (g) |
4.69 ± 0.11 |
3.35 ± 0.07* |
2.50 ± 0.12*# |
0.68 ± 0.06* # $ |
PE (% of KCl) |
45.6 ± 2.1 |
85.7 ± 2.4* |
101.0 ± 4.6* |
167.1 ± 26.1* # |
pD2 to PE |
6.90 ± 0.89 |
7.32 ± 0.03* |
6.88 ± 0.18# |
7. 05 ± 0.08# |
Rmax ACh (%) |
57.0 ± 2.0 |
49.6 ± 2.2 |
43.5± 2.7* |
48.2 ± 3.0 |
pD2 to ACh |
7.06 ± 0.13 |
7.15 ± 0.18 |
7.06 ± 0.11 |
6.53 ± 0.14* # $ |
Rmax SNP (%) |
98.5 ± 0.5 |
99.4 ± 0.4 |
96.0 ± 1.0# |
97.7 ± 1.5$ |
pD2 to SNP |
9.07 ± 0.07 |
8.44 ± 0.05* |
8.87 ± 0.06# |
8.97 ± 0.12# |
Table 3: Quantitative analysis of vascular function.
Data is represented as mean ± SEM. In each group, 7-8 independent experiments (n=23-31 aortic rings/group). * p < 0.05 vs Control; $ p < 0.05 vs Control-IR; # p < 0.05 vs SHRSP. SHRSP indicates spontaneously hypertensive stroke-prone rats and IR, ischemia/reperfusion.
Furthermore, the sensitivity to PE (pD2-value) was increased in the SHRSP rats compared to controls and decreased in the SHRSP-IR rings compared to SHRSP group. In contrast to PE, SHRSP rats showed significantly reduced vasoconstrictive responses to high K+-induced depolarization when compared to controls (Table 3, Figure 2B). IR injury significantly decreased contractile responses to KCl in both control and SHRSP rats compared to the corresponding groups, which was further increased in SHRSP-IR rats compared to control-IR group (Table 3, Figure 2B). Values of maximal relaxation (Rmax, %) and pD2 (-logEC50, EC50 being the concentration of substance that elicited 50% of the maximal response) to the vasorelaxant action of acetylcholine (ACh) and sodium nitroprusside (SNP), and contraction induced by phenylephrine (PE, 10-5M) in thoracic aortic rings.

Figure 2: Ex vivo vascular function. (A) Concentration-response curves for phenylephrine-induced vasocontriction, (B) contractile responses to high K+-induced depolarization, concentration-response curves for (C) acetylcholine-induced endothelium-dependent- and for (D) sodium nitroprusside induced endothelium-independent vasorelaxation of isolated thoracic aortic rings. Data is represented as mean ± SEM. n=23-28 rings from 7-8 rats/group. * p < 0.05 vs Control; $ p < 0.05 vs Control-IR; # p < 0.05 vs SHRSP. SHRSP indicates spontaneously hypertensive stroke-prone rats; IR, ischemia/reperfusion.
3.2.2 Endothelium-dependent vasorelaxation of aortic rings: In aortic rings precontracted with 10-6 M PE, 10-9 - 10-4 M ACh induced a concentration-dependent relaxation (Figure 2C). IR significantly reduced Rmax to ACh in the normoxia control group, whereas unexpectedly, it had no effect on the aortic rings from SHRSP rats. IR significantly reduced the sensitivity of aortic rings to ACh in SHRSP-IR rats compared to controls, SHRSP and control-IR groups (Table 3).
3.2.3 Endothelium-independent vasorelaxation of aortic rings: Figure 2D shows concentration-dependent relaxations induced by 10-10-10-5 M SNP, an endothelium-independent vasodilator. Rmax to SNP was significantly increased in the shrsp-IR group compared to control-IR (Table 3). The concentration-response curve to SNP was significantly shifted to the right in aorta from SHRSP compared to controls and in the SHRSP-IR rats compared to SHRSP group (Table 3, Figure 2D).
3.4.4 Protein expression in the aorta: The densitometric analysis of bands for eNOS showed a tendency toward higher expression in the SHRSP compared to controls and in the SHRSP-IR compared to control-IR rats (Figure 3A). HO-1 protein expression was significantly increased in the SHRSP-IR compared to the control-IR (Figure 3B). HIF-1α protein expression was significantly decreased in the SHRSP-IR rats compared to SHRSP group (Figure 3C).
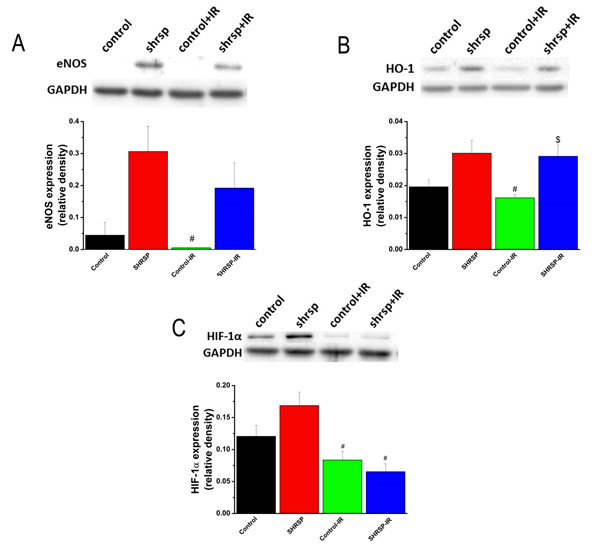
Figure 3: Protein expression in the aorta. Representative western blot analysis of (A) endothelial nitric oxide synthase (eNOS), (B) heme-oxygenase (HO)-1, and (C) hypoxia inducible factor (HIF)-1α. Glyceraldehyde-3-phosphate dehydrogenase (GAPDH), housekeeping protein was used as reference. Data is represented as mean ± SEM. n=4-6 rats/group. * p < 0.05 vs. Control, $ p < 0.05 vs. Control-IR, # p < 0.05 vs. SHRSP. SHRSP indicates stroke-prone spontaneously hypertensive rats and IR ischemia/reperfusion.
4. Discussion
In the present study, we explored the influence of IR on high blood pressure and age induced pathogenic vascular changes, in a model of in vitro vascular IRI. To the best of our knowledge, this is the first paper showing that cold ischemia followed by warm reperfusion in aortas of aged SHRSP with established hypertension increases the susceptibility to smooth muscle contractile impairment. Alteration in HO-1 protein expression may, in part, contribute to the pathological processes. Elderly patients with arterial hypertension are more prone to PAD. Therefore, targeting specific proteins and gaining an understanding of the molecular mechanisms may represent a promising therapeutic approach to reduce the IR injury, and thus the vascular complications, in older hypertensive patients.
4.1 Effects of high blood pressure in aorta of senescent rats
Hypertension is associated with an increased sympathetic tone and changes in vascular responses, such as impaired relaxation or augmented contractility [19,20]. In the present study, combination of arterial hypertension and ageing was associated with an increased contractile function of the VSM aortic rings to α1-adrenergic receptor agonist PE. In contrast to PE, we observed significantly reduced vasocontriction responses to high K+-induced depolarization in aged aorta from hypertensive rats when compared to controls. Possibly, the difference between the reactivity of aortic segments to PE and to KCl may be the consequence of the weaker calcium permeability of the VSM cell membrane in old hypertensive rats compared to old normotensive animals. In these old SHRSP, the VSM cells may have undergone adaptive changes in response to the increased arterial pressure. The increase in vascular reactivity could have been caused by an increase in wall thickness [21]. In essential hypertension, large arterial remodelling is characterized mainly by an increase in intima-media thickness and a lumen enlargement of proximal elastic arteries [22]. When normalized to the body weights, the mean aortic lumen area, wall thickness and area were significantly higher in old SHRSP than in controls. Due to chronic high pressure and ageing, the compliance of the vascular system is reduced (i.e. arterial stiffness is increased), and thus the capacity of modulating flow pulsatility is diminished. The thickening of the intima-media and increased cross-sectional luminal area reflect morphological abnormalities and could contribute to aortic stiffness. HIF-1, a key regulator of the cellular hypoxic response, is involved in different vascular diseases. Inhibition of HIF-1α has been shown to decrease vascular wall thickness in a model of carotid stenosis in rats.23 In the present study, the tendency toward an increase in HIF-1α protein expression in the aorta of SHRSP may be involved in vascular remodelling. Additionally, with ageing and elevated mean blood pressure, an enlargement of proximal elastic arteries has been extensively described in humans [23,24]. This is generally attributed to the fragmentation of the load-bearing component, such as elastin fibers, from pulsatile wall stress, ultimately leading to fatigue and failure of elastin within the vessel wall. However, cellular growth and apoptosis, low-grade inflammation, and fibrosis of VSM cells could also be involved [25]. It is well known that endothelial cells produce nitric oxide (NO), prostacyclin (PGI2), and endothelium-derived hyperpolarizing factor (EDHF) regulating the vascular tone. Endothelial function was evaluated ex vivo by performing ACh concentration-response curves in PE-precontracted isolated aortic rings [26-29]. In the present study, hypertension had slight effect on endothelium-dependent relaxation in SHRSP compared with that in normotensive rats. Although we did not examine endothelial NO production, tendency toward an increase in protein expression of eNOS was observed in the thoracic aorta from SHRSP. It is tempting to speculate that the enhanced eNOS expression is an adaptive response related to the increase in superoxide anion generation. It has been shown that this genetic model of hypertension (i.e. SHRSP rats) was associated with an increase in vascular superoxide production, which could contribute to the decreased availability of basal NO [30]. Additionally, Imai et al. have showed that transgenic overexpression of HO-1 in VSM cells leads to an elevation of arterial pressure and aortic rings exhibited a reduction of NO-mediated relaxing responses [31]. In line with these observations, the protein expression of HO-1, an important modulator of blood pressure, showed a tendency to be increased in the aorta of SHRSP, suggesting its involvement in vascular endothelial dysfunction. The nitrodilator SNP releases NO spontaneously and acts directly on the VSM, leading to the relaxation of the muscle cells, thus inducing blood vessels dilatation. SNP has most commonly been used to assess the vascular responsiveness as endothelial-independent vasodilator. In the present study, in older hypertrophic aorta the concentration-response curves for the SNP were significantly shifted rightwards compared to the senescent group.
4.2 Effects of IR injury in aorta of older hypertensive rats
To the best of our knowledge, this is the first study that shows significant alterations of vascular reactivity by IR in old hypertensive rats. In the present work, we demonstrated that augmented α1-receptor-mediated contractions induced by PE in SHRSP aorta was further increased by IR. During IR, reactive oxygen species produced by VSM cells have been reported to be responsible for the impaired adrenergic function in aortic rings. The release of calcium from the sarcoplasmic reticulum constitutes the major defect in hypoxia/reoxygenation induced vasoconstrictive dysfunction [32,33]. Additionally, we showed that high K+-induced contraction was further reduced in SHRSP aorta subjected to IR, indicating smooth muscle damage. The lack of oxygen has multiple effects modulating the vascular system. The main hypoxia sensors at the cellular levels are HIF and HO. It has been shown that HIF-1α, a key factor for responses to low oxygen, seems to play an important role in the regulation of VSM cell contraction induced by hypoxia, by regulating eNOS, COX-2 and HO-1 expression [34]. In the present study, our western blot results showed that IR down-regulated HIF-1α in the both normotensive and hypertensive rats, suggesting its detrimental role in vascular IR injury. Furthermore, the impaired vascular reactivity in the ischemic/reperfused aortic segments could be related to the marked increase in the protein expression of eNOS, evidenced by our western blot analysis. Additionally, HO-1 expression can be increased by various stress factors including IR. In the present study, its expression in the aorta from SHRSP submitted to IR was increased when compared to the control-IR group. Some limitations need to be addressed. The vascular reactivity of the isolated thoracic aortic rings was studied ex vivo in a standard tissue bath system. Translation of these findings into meaningful in vivo conditions should be confirmed with the involvement of non-aortic tissue, blood flow to the tissues, and leucocytes activation. Even though it is not common in clinical practice, storage at 4°C for 24h is a well-established in vitro vascular model for IR injury. Additionally, further studies are required to confirm the effects of IR injury on peripheral arteries of the legs. In conclusion, both structural changes and alteration in HO protein expression, in part, may contribute to the pathological processes associated with the impairment in vascular contractile function in response to IR from old hypertensive rats. Collectively, targeting specific proteins and understanding molecular mechanisms may be a promising and therapeutically feasible approach to protect elderly hypertensive patients with PAD.
Acknowledgments
This study was supported by the Land Baden-Württemberg, Germany, by the Medical Faculty of the University of Heidelberg, Germany (to S. Korkmaz-Icöz), by the János Bolyai Research Scholarship of the Hungarian Academy of Sciences (to T. Radovits), and by a grant from the National Research, Development and Innovation Office (NKFIH) of Hungary (NVKP_16-1-2016-0017). The excellent technical assistance of Tobias Mayer, Karin Sonnenberg and Lutz Hoffmann is greatly acknowledged. The authors also thank Dr. Xiaoxin Sun and Dr. Kunsheng Li for histological studies.
Conflict of Interest
None.
References
- Hearse DJ and Bolli R. Reperfusion induced injury: manifestations, mechanisms, and clinical relevance. Cardiovascular research 13 (1992): 101-108.
- Tan JL and Thakur K. Systolic Hypertension StatPearls Treasure Island (FL) (2021).
- Bohr DF. Reactivity of vascular smooth muscle from normal and hypertensive rats: effect of several cations. Federation proceedings (1974): 127-132.
- Marin J. Age-related changes in vascular responses: a review. Mechanisms of ageing and development 12 (1995): 71-114.
- Radovits T, Seres L, Gero D, et al. Single dose treatment with PARP-inhibitor INO-1001 improves aging-associated cardiac and vascular dysfunction. Experimental gerontology 6 (2007): 676-685.
- Radovits T, Seres L, Gero D, et al. The peroxynitrite decomposition catalyst FP15 improves ageing-associated cardiac and vascular dysfunction. Mechanisms of ageing and development 5 (2007): 173-181.
- Okamoto K and Aoki K. Development of a strain of spontaneously hypertensive rats. Japanese circulation journal 11 (1963): 282-293.
- Yamori Y, Igawa T, Kanbe T, et al. Mechanisms of structural vascular changes in genetic hypertension: analyses on cultured vascular smooth muscle cells from spontaneously hypertensive rats. Clin Sci (Lond) (1981): 121s-123s.
- Pinto YM, Paul M and Ganten D. Lessons from rat models of hypertension: from Goldblatt to genetic engineering. Cardiovascular research 23 (1998): 77-88.
- Yamori Y. Implication of hypertensive rat models for primordial nutritional prevention of cardiovascular diseases. Clinical and experimental pharmacology & physiology 4 (1999): 568-572.
- Graham D, Hamilton C, Beattie E, et al. Comparison of the effects of omapatrilat and irbesartan/hydrochlorothiazide on endothelial function and cardiac hypertrophy in the stroke-prone spontaneously hypertensive rat: sex differences. Journal of hypertension 6 (2004): 329-337.
- Barnucz E, Veres G, Hegedus P, et al. Prolyl-hydroxylase inhibition preserves endothelial cell function in a rat model of vascular ischemia reperfusion injury. The Journal of pharmacology and experimental therapeutics 9 (2013): 25-31.
- Radovits T, Lin LN, Zotkina J, et al. Endothelial dysfunction after long-term cold storage in HTK organ preservation solutions: effects of iron chelators and N-alpha-acetyl-L-histidine. J Heart Lung Transplant 12 (2008): 208-216.
- Veres G, Hegedus P, Barnucz E, et al. Addition of vardenafil into storage solution protects the endothelium in a hypoxia-reoxygenation model. Eur J Vasc Endovasc Surg 4 (2013): 242-248.
- Korkmaz S, Loganathan S, Mikles B, et al. Nitric oxide- and heme-independent activation of soluble guanylate cyclase attenuates peroxynitrite-induced endothelial dysfunction in rat aorta. Journal of cardiovascular pharmacology and therapeutics 11 (2013): 70-77.
- Korkmaz-Icoz S, Radovits T, Loganathan S, et al. Prolonging hypothermic ischaemic cardiac and vascular storage by inhibiting the activation of the nuclear enzyme poly(adenosine diphosphate-ribose) polymerase. Eur J Cardiothorac Surg 34 (2017): 829-835.
- Korkmaz-Icoz S, Vater A, Li S, et al. Mild type 2 diabetes mellitus improves remote endothelial dysfunction after acute myocardial infarction. Journal of diabetes and its complications 6 (2015): 1253-1260.
- Dileepan K N, Johnston T P, Li Y, et al. Deranged aortic intima-media thickness, plasma triglycerides and granulopoiesis in Sl/Sl(d) mice. Mediators of inflammation 7 (2004): 335-341.
- Marin J. Mechanisms involved in the increased vascular resistance in hypertension. J Auton Pharmacol 5 (1993): 127-176.
- Mattei P, Virdis A, Ghiadoni L, et al. Endothelial function in hypertension. J Nephrol 6 (1997): 192-197.
- Folkow B. The haemodynamic consequences of adaptive structural changes of the resistance vessels in hypertension. Clin Sci 7 (1971): 1-12.
- Benetos A, Laurent S, Hoeks AP, et al. Arterial alterations with aging and high blood pressure. A noninvasive study of carotid and femoral arteries. Arteriosclerosis and thrombosis: a journal of vascular biology / American Heart Association 8 (1993): 90-97.
- Lambert CM, Roy M, Robitaille GA, et al. HIF-1 inhibition decreases systemic vascular remodelling diseases by promoting apoptosis through a hexokinase 2-dependent mechanism. Cardiovascular research 10 (2010): 196-204.
- Lam CS, Xanthakis V, Sullivan LM, et al. Aortic root remodeling over the adult life course: longitudinal data from the Framingham Heart Study. Circulation 8 (2010): 884-890.
- Intengan HD and Schiffrin EL. Vascular remodeling in hypertension: roles of apoptosis, inflammation, and fibrosis. Hypertension 13 (2001): 581-587.
- Furchgott RF and Vanhoutte PM. Endothelium-derived relaxing and contracting factors. Faseb J 9 (1989): 2007-2018.
- Ignarro LJ, Buga GM, Wood KS, et al. Endothelium-derived relaxing factor produced and released from artery and vein is nitric oxide. Proceedings of the National Academy of Sciences of the United States of America (1987): 9265-9269.
- Moncada S, Gryglewski R, Bunting S, et al. An enzyme isolated from arteries transforms prostaglandin endoperoxides to an unstable substance that inhibits platelet aggregation. Nature 14 (1976): 663-665.
- Griffith TM. Endothelium-dependent smooth muscle hyperpolarization: do gap junctions provide a unifying hypothesis? British journal of pharmacology 16 (2004): 881-903.
- Kerr S, Brosnan MJ, McIntyre M, et al. Superoxide anion production is increased in a model of genetic hypertension: role of the endothelium. Hypertension 11 (1999): 1353-1358.
- Imai T, Morita T, Shindo T, et al. Vascular smooth muscle cell-directed overexpression of heme oxygenase-1 elevates blood pressure through attenuation of nitric oxide-induced vasodilation in mice. Circulation research 9 (2001): 55-62.
- Bauersachs J, Bouloumie A, Mulsch A, et al. Vasodilator dysfunction in aged spontaneously hypertensive rats: changes in NO synthase III and soluble guanylyl cyclase expression, and in superoxide anion production. Cardiovascular research 12 (1998): 772-779.
- Benoit JN and Taylor MS. Vascular reactivity following ischemia/reperfusion. Front Biosci 18 (1997): e28-33.
- Zhang Y, Liu LM, Ming J, et al. [Regulatory role of hypoxia inducible factor-1 alpha in the changes of contraction of vascular smooth muscle cell induced by hypoxia]. Zhongguo wei zhong bing ji jiu yi xue = Chinese critical care medicine = Zhongguo weizhongbing jijiuyixue 11 (2007): 647-651.