Rectus Femoris Muscle Elasticity and Stiffness Correlates with Maximal Oxygen Consumption in Triathletes
Article Information
Georg Gavronski1, Ain Reimets1, Jaak Talts1, Indrek Koovit2, Tõnis Mandel3, Ragnar Viir4, Eero Vasar1, Alar Veraksitš1*
1Chair of Physiology, Institute of Biomedicine and Translational Medicine, Faculty of Medicine, University of Tartu, 19 Ravila Street, 50411 Tartu, Estonia
2Radiology Clinic, Tartu University Hospital, L. Puusepa 8, G.1.R, 51014 Tartu
3Sports Medicine and Rehabilitation Clinic, Tartu University Hospital, L. Puusepa 1a, 50406 Tartu
4Ragnar Viir Limited Partnership, Sorsavuorenkatu 8 B 41, 00810 Helsinki, Finland
*Corresponding Author: Alar Veraksitš, Chair of Physiology, Institute of Biomedicine and Translational Medicine, Faculty of Medicine, University of Tartu, 19 Ravila Street, 50411 Tartu, Estonia.
Received: 15 December 2023; Accepted: 22 December 2023 2023; Published: 05 January 2024
Citation: Georg Gavronski, Ain Reimets, Jaak Talts, Indrek Koovit, Tõnis Mandel, Ragnar Viir, Eero Vasar, Alar Veraksitš. Rectus Femoris Muscle Elasticity and Stiffness Correlates with Maximal Oxygen Consumption in Triathletes. Journal of Orthopedics and Sports Medicine. 6 (2024): 01-11.
View / Download Pdf Share at FacebookAbstract
VO2max is considered single best indicator of cardiovascular fitness and aerobic endurance. We analyzed retrospectively, are there any relationships between muscle parameters and oxygen consumption in a study where the myoton equipment was used to establish muscle biomechanical properties, such as elasticity, stiffness, and tension (measured as oscillation frequency) in triathletes. Eight muscles were studied in 14 male triathletes over three years. Relaxed and contracted states of muscles were measured. VO2max was recorded in these athletes up to four times during this period. Average values were calculated for each athlete and High (max 71.8–min 62.3 ml/ kg/min) and Low (59.1–51.3) oxygen consumption groups were formed. Higher oxygen consumption correlated significantly (r=-0.58; p=0.029) with improved elasticity (represented by smaller decrement values) of the rectus femoris muscle in a contracted state. Also, in the High VO2max group, this muscle (in a relaxed state) was significantly more elastic and stiffer at the same time compared to the Low group. An ultrasound registration was also conducted to observe the depth of the device's impact in the posterior crural muscles. It was confirmed that deep and substantial tissue disturbances were caused by this impact. According to our findings, myotonometry is an adequate method to establish muscle parameters. Elasticity and stiffness of the rectus femoris muscle may determine success in triathlon.
Keywords
Muscle elasticity; Oxygen consumption; Triathletes; Ultrasound
Sports medicine articles Sports medicine Research articles Sports medicine review articles Sports medicine PubMed articles Sports medicine PubMed Central articles Sports medicine 2023 articles Sports medicine 2024 articles Sports medicine Scopus articles Sports medicine impact factor journals Sports medicine Scopus journals Sports medicine PubMed journals Sports medicine medical journals Sports medicine free journals Sports medicine best journals Sports medicine top journals Sports medicine free medical journals Sports medicine famous journals Sports medicine Google Scholar indexed journals Fascia tissue articles Fascia tissue Research articles Fascia tissue review articles Fascia tissue PubMed articles Fascia tissue PubMed Central articles Fascia tissue 2023 articles Fascia tissue 2024 articles Fascia tissue Scopus articles Fascia tissue impact factor journals Fascia tissue Scopus journals Fascia tissue PubMed journals Fascia tissue medical journals Fascia tissue free journals Fascia tissue best journals Fascia tissue top journals Fascia tissue free medical journals Fascia tissue famous journals Fascia tissue Google Scholar indexed journals Rehabilitation articles Rehabilitation Research articles Rehabilitation review articles Rehabilitation PubMed articles Rehabilitation PubMed Central articles Rehabilitation 2023 articles Rehabilitation 2024 articles Rehabilitation Scopus articles Rehabilitation impact factor journals Rehabilitation Scopus journals Rehabilitation PubMed journals Rehabilitation medical journals Rehabilitation free journals Rehabilitation best journals Rehabilitation top journals Rehabilitation free medical journals Rehabilitation famous journals Rehabilitation Google Scholar indexed journals Oxygen articles Oxygen Research articles Oxygen review articles Oxygen PubMed articles Oxygen PubMed Central articles Oxygen 2023 articles Oxygen 2024 articles Oxygen Scopus articles Oxygen impact factor journals Oxygen Scopus journals Oxygen PubMed journals Oxygen medical journals Oxygen free journals Oxygen best journals Oxygen top journals Oxygen free medical journals Oxygen famous journals Oxygen Google Scholar indexed journals Muscle articles Muscle Research articles Muscle review articles Muscle PubMed articles Muscle PubMed Central articles Muscle 2023 articles Muscle 2024 articles Muscle Scopus articles Muscle impact factor journals Muscle Scopus journals Muscle PubMed journals Muscle medical journals Muscle free journals Muscle best journals Muscle top journals Muscle free medical journals Muscle famous journals Muscle Google Scholar indexed journals Athletes articles Athletes Research articles Athletes review articles Athletes PubMed articles Athletes PubMed Central articles Athletes 2023 articles Athletes 2024 articles Athletes Scopus articles Athletes impact factor journals Athletes Scopus journals Athletes PubMed journals Athletes medical journals Athletes free journals Athletes best journals Athletes top journals Athletes free medical journals Athletes famous journals Athletes Google Scholar indexed journals Physical exercise articles Physical exercise Research articles Physical exercise review articles Physical exercise PubMed articles Physical exercise PubMed Central articles Physical exercise 2023 articles Physical exercise 2024 articles Physical exercise Scopus articles Physical exercise impact factor journals Physical exercise Scopus journals Physical exercise PubMed journals Physical exercise medical journals Physical exercise free journals Physical exercise best journals Physical exercise top journals Physical exercise free medical journals Physical exercise famous journals Physical exercise Google Scholar indexed journals Achilles tendon articles Achilles tendon Research articles Achilles tendon review articles Achilles tendon PubMed articles Achilles tendon PubMed Central articles Achilles tendon 2023 articles Achilles tendon 2024 articles Achilles tendon Scopus articles Achilles tendon impact factor journals Achilles tendon Scopus journals Achilles tendon PubMed journals Achilles tendon medical journals Achilles tendon free journals Achilles tendon best journals Achilles tendon top journals Achilles tendon free medical journals Achilles tendon famous journals Achilles tendon Google Scholar indexed journals Muscle stiffness articles Muscle stiffness Research articles Muscle stiffness review articles Muscle stiffness PubMed articles Muscle stiffness PubMed Central articles Muscle stiffness 2023 articles Muscle stiffness 2024 articles Muscle stiffness Scopus articles Muscle stiffness impact factor journals Muscle stiffness Scopus journals Muscle stiffness PubMed journals Muscle stiffness medical journals Muscle stiffness free journals Muscle stiffness best journals Muscle stiffness top journals Muscle stiffness free medical journals Muscle stiffness famous journals Muscle stiffness Google Scholar indexed journals Triathletes articles Triathletes Research articles Triathletes review articles Triathletes PubMed articles Triathletes PubMed Central articles Triathletes 2023 articles Triathletes 2024 articles Triathletes Scopus articles Triathletes impact factor journals Triathletes Scopus journals Triathletes PubMed journals Triathletes medical journals Triathletes free journals Triathletes best journals Triathletes top journals Triathletes free medical journals Triathletes famous journals Triathletes Google Scholar indexed journals Superficial tissue articles Superficial tissue Research articles Superficial tissue review articles Superficial tissue PubMed articles Superficial tissue PubMed Central articles Superficial tissue 2023 articles Superficial tissue 2024 articles Superficial tissue Scopus articles Superficial tissue impact factor journals Superficial tissue Scopus journals Superficial tissue PubMed journals Superficial tissue medical journals Superficial tissue free journals Superficial tissue best journals Superficial tissue top journals Superficial tissue free medical journals Superficial tissue famous journals Superficial tissue Google Scholar indexed journals Biceps brachii articles Biceps brachii Research articles Biceps brachii review articles Biceps brachii PubMed articles Biceps brachii PubMed Central articles Biceps brachii 2023 articles Biceps brachii 2024 articles Biceps brachii Scopus articles Biceps brachii impact factor journals Biceps brachii Scopus journals Biceps brachii PubMed journals Biceps brachii medical journals Biceps brachii free journals Biceps brachii best journals Biceps brachii top journals Biceps brachii free medical journals Biceps brachii famous journals Biceps brachii Google Scholar indexed journals Gastrocnemius muscle articles Gastrocnemius muscle Research articles Gastrocnemius muscle review articles Gastrocnemius muscle PubMed articles Gastrocnemius muscle PubMed Central articles Gastrocnemius muscle 2023 articles Gastrocnemius muscle 2024 articles Gastrocnemius muscle Scopus articles Gastrocnemius muscle impact factor journals Gastrocnemius muscle Scopus journals Gastrocnemius muscle PubMed journals Gastrocnemius muscle medical journals Gastrocnemius muscle free journals Gastrocnemius muscle best journals Gastrocnemius muscle top journals Gastrocnemius muscle free medical journals Gastrocnemius muscle famous journals Gastrocnemius muscle Google Scholar indexed journals VO2max articles VO2max Research articles VO2max review articles VO2max PubMed articles VO2max PubMed Central articles VO2max 2023 articles VO2max 2024 articles VO2max Scopus articles VO2max impact factor journals VO2max Scopus journals VO2max PubMed journals VO2max medical journals VO2max free journals VO2max best journals VO2max top journals VO2max free medical journals VO2max famous journals VO2max Google Scholar indexed journals Thigh muscles articles Thigh muscles Research articles Thigh muscles review articles Thigh muscles PubMed articles Thigh muscles PubMed Central articles Thigh muscles 2023 articles Thigh muscles 2024 articles Thigh muscles Scopus articles Thigh muscles impact factor journals Thigh muscles Scopus journals Thigh muscles PubMed journals Thigh muscles medical journals Thigh muscles free journals Thigh muscles best journals Thigh muscles top journals Thigh muscles free medical journals Thigh muscles famous journals Thigh muscles Google Scholar indexed journals
Article Details
1. Introduction
The structural proteins of muscle filaments and the corresponding fascia tissue are contributing to the biomechanical properties of muscles themselves and myofascial structures in general [1-3]. Kyröläinen et al. [4] observed the lower-mobility titin band only in the most economical runner in a small group of sprinters. According to Heyward [5], at age 20–29 the level of superior oxygen consumption among general population in men is >56 ml/kg/min. It has been suggested that VO2max 65 ml/min/kg separates the top-level endurance runners from the rest [6]. On the other hand, it would be logical to assume that if the locomotion of an athlete is exceptionally economic due to the beneficial muscle composition and properties, the value of maximal oxygen consumption may not be enormously high.
Economy of locomotion could serve as a predictor of athletic ability, and if this is the case, the biomechanical parameters of muscles must play a role. Muscle properties have been shown to differ among highly and less trained athletes [7,8]. Stiffness has been found to contribute strongly to the efficacy of the athlete’s performance [9-11]. However, this is not always the case [12]. Muscle stiffness has been reported to differ according to performance ability, and it has been suggested that stiffer muscles allow cyclists to perform better on certain occasions [13]. Dumke et al. [14] demonstrated that muscle stiffness is related to running economy at a speed that approximates endurance competition. Myotonometric research show biomechanical properties correlating to aspects of muscles’ function and competition results [15-17]. Jiménez-Sánchez et al. [18] found, that passive resistive torque of the triceps surae muscle (measured with the isokinetic device) is higher in more stiff, tense and elastic muscle.
During triathlon competitions, running performance seems to be extremely important [19]. Triathletes differ in their parameters from pure endurance runners by larger muscle mass necessary for cycling and swimming [20], though. Bonacci et al. [21,22] showed that switching from cycling to running is a crucial neuromuscular event that ensures success and singles out the elite from the rest.
Elasticity of specific muscles is defined less in literature. It is a quality describing the ability of the body to resume the initial form after deformation by compression, stretching, twisting or bending. Industrial elastic springs are made from stiff and plastic materials. Cavagna et al. [23] suggested that elastic energy is stored and released in muscles and tendons during sprints. In wallabies and kangaroos, elastic energy may account for up to half of the performed work at hopping without additional energy cost [24]. Elasticity is usually mentioned in studies using elastography—detection of share-wave propagation in tissue(s)—, but this propagation depends on the arrangements of the muscular structures [25-27]. In our study a different method and device was used and biomechanical properties like elasticity, stiffness, and the tension in the muscle were established. Ditroilo et al. [28] showed that elasticity depends on the stretch and that muscles become slacker/more plastic when less stretched. Jarocka et al. [29] showed that if the contraction force rises gradually, muscle elasticity does not behave accordingly. Instead, it rises rapidly at a low contraction force to a high plateau level while stiffness and tension correlates almost linearly with the generated power. It is important to note that all three parameters are calculated from the same single measurement. According to most recent observations, the elasticity of the muscles in master athletes is lower than in sedentary coevals [30] and intensive physical exercise significantly increases stiffness in the Achilles tendon [31]. Most recent work correlations strongly Achilles tendon elasticity (among other parameters) to countermovement jump height [32].
As the impact from the myoton device is light and given on the skin, there are questions about its ability to reach and describe muscle properties. We quote, “So it is possible that during rest conditions, the Myoton-3 measures mainly the oscillations of the skin and subcutaneous tissue which are provoked by its testing-end.” [29]; “Myotonometry is quick and inexpensive, but tends to be superficial or merely qualitative.” [27]. On the other hand, “The linear relationship with force output suggested that the device was giving a valid recording of the viscoelastic stiffness of the muscle rather than that of the subcutaneous tissue.” [33]. This is confirmed by Zinder and Padua [34], and using newer myoton device by Kelly et al. [35], and Li et al. [36]. A reliability study made by Bravo-Sánchez et al. [37] revealed that the thicknesses of all tissues beneath the testing-end (muscle tissue, connective and superficial tissue, adipose tissue) correlated separately with the myoton registered stiffness values. Fröhlich-Zwahlen et al. [38] obtained analogues results previously. As the effect of the device on tissues was not clear, we decided to study this using an ultrasound (US) registration that allows to detect deep tissue disturbances [26]. This experiment was conducted to answer a single question—does the 0.4 N mechanical impact from the device reach the muscle tissue—as it has not been studied so far.
2. Methods
2.1 Atheletes
The study protocol was approved by The Ethics Review Committee on Human Research of the University of Tartu (No. 94/17; 21.05.2001 for athletes and 242/T-21 for the US study). All participants gave their informed consent in writing. Fourteen male triathletes were observed during a period of 36 months. Athletes of the national junior team were studied from whom most had been trained regularly as triathletes under the same coach. Elder triathletes were high rank hobby athletes. The criterion was set that the athlete must have been highly active more than 3 years to be chosen into the study. Their height was from 176 to 192 cm, and their body mass varied from 66.1 to 81.6 kg, age varied between 17–41 years at the beginning of the study. Average values of height, weight and BMI were used.
During the study period, the athletes were tested on VO2max up to 4 times. According to their average VO2max results, the group was divided into half.
Separately, three non-athletes but athletic men with BMIs 27, 26, and 23, were used for the US study.
2.2 Myotonometry
The testing-end of the device with the effective weight P, is placed on the skin surface transversally to the muscle (level S as dotted line; Figure 1B) compressing the tissue in terms of ΔS. The connected to testing-end electromagnetic driver (Figure 1A) is fired (time-point t1) producing a short impulse (tk=15ms=t1-t2) terminating with a quick release at moment t2. This generates a mechanical force of 0.4 N (Newton). The device then monitors evoked primary oscillatory waves, as the testing-end stays in contact with tissue surface. Acceleration of the testing-end is recorded at a 3 KHz frequency.

Figure 1: Myotonometry [with courtesy to Dr (Habil. Biol.) PhD Arved Vain]. A) Working principle of Myoton models 2 and 3. B) Schematic graphs and formulas (see the text). Waveforms of displacement (s), velocity (v), and acceleration (a). C) Graphic presentation of the acceleration values recorded during the measurement.
Elasticity is the ability of a tissue to restore its initial shape and is characterized by the logarithmic decrement of the damped oscillations (Equation 1). The smaller the value, the more elastic is the tissue showing that less energy is lost in each following oscillation. Stiffness reflects the resistance of the tissue to the force that changes its shape (Equation 2). The equipment detects it with the testing-end during the initial impact. The higher the value, the stiffer is the tissue, showing that more energy is needed to modify muscle shape. The frequency of the damped oscillations (Equation 3) characterizes the state of the tissue – the higher the value, the more tense is the tissue. We used Myoton-2 on athletes and Myoton-3 in the US study. The working principle and build-up of both models is the same (Figure 1A).
2.3 Procedures
Muscles: BB – biceps brachii (caput longum); TB–triceps brachii (caput longum); BF–biceps femoris (caput longum); RF–rectus femoris; TA–tibialis anterior; GC–gastrocnemius (caput mediale); LD–latissimus dorsi; PM–pectoralis major (pars sternocostalis) were measured bilaterally in both relaxed and contracted state while the subjects rested supine or prone, depending on the muscles measured, on a portable massage table. All the measurements were carried out by the same person. The measuring point was marked on the skin at the most prominent point of the muscle belly at contraction [39]. Contraction was standardized simply by the same position of the limb and additional weight (a 2.3 kg dumbbell) was used when the muscles of the upper body and the brachium were measured. A heavier weight caused muscle tremor which disrupted the measurements. To evoke contraction in the muscles of the brachium (BB and TB), the subject was holding his arm at the level of the shoulder and raised it to an angle of 45° from the horizontal axis (measured with a fixed angle), holding a dumbbell. To contract the PM muscle (supine position), the subject stretched out his arm horizontally on the side over the table edge, holding a dumbbell in his hand. For LD (prone position), the subject stretched his arm similarly over his head. The contraction of the BF and the TF muscles was evoked by raising the leg to an angle of 45°. To contract the GC and the TA, the maximum tarsal dorsiflexion and plantarflexion were performed against the fixed table. Most measurements were taken weekly on Wednesday mornings before the training sessions. There were few periods when measurements were taken daily for 3–5 days, also in a field situation before the warm-up for the competition and immediately after the competition. In two longer periods from summer to winter (about five months), no measurements were taken.
The subjects were tested for VO2max up to four times during this period. Standardized complex laboratory tests of functional aerobic capability were performed on a Technogym Runrace HC 1400 (Italy) treadmill. The same gradually increasing load regime was used. After 5 min warm up at speed 8km/h, the starting speed was set to 10km/h and then raised by 2km/h after every 3 minutes up to 14 km/h. From that point, the speed was raised in the same rhythm by 1 km/h until exhaustion. Respiration parameters with O2 and CO2 fractions in the exhaled air were measured using a True Max 2400 (Parvo Medics, USA) computerized complex. The criteria used to confirm that VO2max had been reached was the achievement of the plateau level in O2 uptake with increase in work-rate at the R value greater than 1,1. The group was divided into half according to average oxygen consumption, seven subjects in both groups. Those in the higher group were marked as ‘High’ and the others as ‘Low’.
2.4 Ultrasound Measurement
We made US recordings on the Musculus gastrocnemius (GC) in the same direction as the mechanical impact from myoton. This muscle was chosen because it is easily accessible. Due to the unavailability of the same device used on athletes and corresponding Myoton-2 models, we used similar but newer Myoton-3 with same build-up and impact force, for that purpose (Figure 1 A,B). The use of Myoton-3 was also reasoned by the fact that prior to MyotonPRO, this model was mainly used.
An ultrasonic apparatus General Electric LOGIQ E9 with an electronic linear array probe 11L (11 MHz wave frequency with 45 mm scanning length) was used to obtain images of the lateral GC muscle area, which were recorded to a video file at 25 Hz. We placed the US inducer on the ventral surface of the GC along the longitudinal vector of the leg on the belly of the muscle and applied impact along the same line with the US beam. The location of the test-end aside the US probe is marked with an arrow in Figure 2. The location of the measuring point was chosen as homogenous as possible, in the area of the lowest pulsation from vessels registered previously by Color-Doppler.
2.5 Image Analysis
To confirm the visual findings, we used Matlab 5.3 software and analyzed recorded video material separating frames from the black and white video clips obtained from the US recording. Extracted frames were divided into pixels on X (768 dots per image) / Y (576 dpi) scale and each dot was monitored on four sequential images establishing the standard deviation of the change on the dark/light scale. New images were generated visualizing the deviations of each monitored dot and higher deviation is presented with the brighter white color (Figure 2).

Figure 2: Illustrative placement of devices to receive the ultrasound recording. Tissue reaction on impact. A) An original image from US video; B) Results of image analysis with MatLab before the impact; C) immediate effect of the impact. White arrow–impact site. Brighter white color on image B-C presents stronger disturbances. Upper guideline marks the superficial aponeurosis of the gastrocnemius muscle, lower guideline marks the depth of 4.5 cm in the tissues.
2.6 Statistics
The Statistica software (version 8; StatSoft Ltd, Bedford, UK) was used. ANOVA was used to compare the general parameters like height, weight, BMI, age and VO2max. The values of the biomechanical parameters in relaxed and contracted states were analyzed separately. According values were pooled either for one person to receive the average “general” value of each biomechanical parameter, or for each muscle. These average values were correlated with VO2max. To compare the muscles between both groups, both sided muscles were considered separately. Non-parametric Mann-Whitney U-test (MW) was used to compare the biomechanical parameters and Spearman Correlation R was also calculated.
3. Results
3.1 General Parameters
The age of the athletes did not differ between the groups (Table 1 shows the age at the end of the study; ANOVA F(1,12)=0.13; p=0.728). There was a significant difference in the height and VO2max values between both groups (Table 1). The general biomechanical parameters of muscles did not differ between the groups. The VO2max did not in correlate with body mass, BMI, age, or height.
Group |
Height (cm) |
Body mass (kg) |
BMI |
Age (years) |
O2 ml/kg/min |
High |
188.7±3.8* |
78.8±11.3 |
22.0±2.5 |
24.4±5.4 |
66.4±3.1** |
Low |
182.0±6.7 |
75.5±7.7 |
22.7±1.8 |
25.9±9.1 |
59.5±2.9 |
Mean±SD are given. N=7 for each group. Age is given at the study’s end year. ANOVA was used and *p<0.05, **p<0.0001 compared to the Low VO2max group are presented |
Table 1: General parameters.
3.2 Image Analysis from the Ultrasound Recordings
The answer to our study question was that the 0.4 N impact from the myoton device is sufficient to reach the muscles through the skin and subcutaneous tissue causing substantial tissue disturbances in muscles. Representative image is presented as Figure 2C.
3.3 Elasticity
Elasticity improves as the decrement value lowers, hence, if the other parameter grows, the negative correlation reflects better elasticity and if both parameters grow, positive correlation reflects lesser elasticity or higher plasticity in turn. For this analysis we calculated single general value for each athlete (N=14). General decrement value in a relaxed state showed higher plasticity at higher body weight and BMI (weight R=0.73, p=0.003; BMI R=0.86, p<0.0001). Oxygen consumption did not correlate with general elasticity while did with the elasticity of the contracted RF muscle (R=-0.58; p=0.029).
To compare between the High and the Low groups, each muscle was considered separately (N=2×7=14 muscles in both groups). A significant difference was revealed in the average elasticity of the RF in both relaxed (MW test; p=0.035) and contracted (p<0.001) state (Figure 3; Table 2).
![]() |
Table 2: Biomechanical parameters of skeletal muscles.
In a relaxed state, the RF muscle was significantly more elastic in High group on both sides (left side: High 1.16±0.13 v. Low 1.78±0.20, p=0.002, N=7; right side: High 1.31±0.18 v. Low 1.71±0.22; p=0.002; N=7). No difference between the groups was observed for contraction (left side: High 1.07±0.2 v. Low 1.28±0.18, p=0.06, N=7; right side: High 1.14±0.17 v. Low 1.27±0.16, p=0.18, N=7).
Additionally, the LD muscle in a contracted state showed a significant difference between the groups (p=0.043; Figure 3; Table 2), but showed no correlation with oxygen consumption.
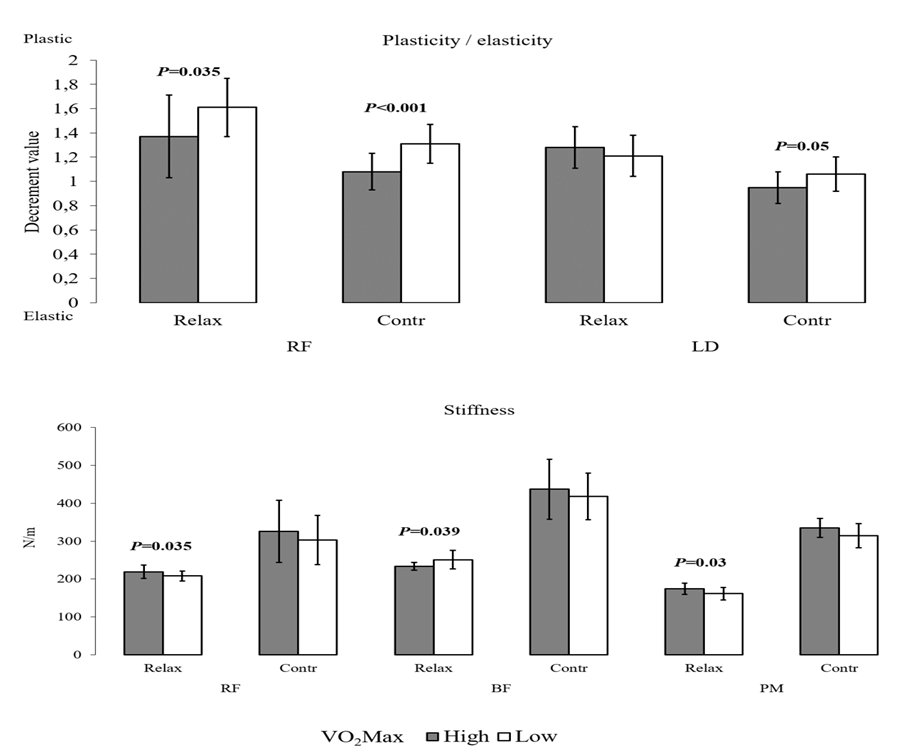
Figure 3: Elasticity and stiffness in significantly different muscles. Differences in muscle biomechanical parameters according to VO2max. Exact data is presented in Table 2.
3.4 Stiffness and Frequency
Both general parameters correlated well with body height (Height/Freq R=0.68, p=0.008; Height/Stiffness R=0.72, p=0.004; N=14) but no difference was evident when taller High VO2max group was compared to the shorter Low group.
Between the two groups, three muscles showed a significant difference in relaxed state and only in stiffness—both thigh muscles (BF p=0.039 and RF p=0.035) and PM (p=0.03; Table 2). Interestingly, the antagonistic thigh muscles showed the opposite behavior—RF was stiffer in the High group and BF in the Low group.
4. Discussion and Conclusions
To our knowledge, this is the first study on the actual tissue impact of myotonometry in general and Myoton-3 device in particular. We stress that the aim of the US experiment was simply to assess the adequacy of a 0.4 N mechanical impact to reach the muscles. As a result, we observed deep tissue disturbances as the impact penetrated the skin and subcutaneous tissues in a relaxed state of the muscle. Our conclusion was supported by the finding that these strong disturbances were observable on 11 consecutive newly generated images that makes the duration of the impact ≈ 440 ms (1000 ms / 25 frames per second x 11 frames), which is consistent with the approximate duration of strong oscillations (Figure 1C). It was not our aim in this study to match the results from other measurement with the findings on video-recordings, neither to make any quantitative analysis nor find any correlations in this matter. These findings are also in same line with our jet unpublished data. According to our findings we can firmly state, that the device is appropriate for describing specifically muscle properties. This was suggested already by Bizzini and Mannion [33]. It is our suggestion that this is possible due to the incompressible nature of soft-tissues consisting mainly of water [1] allowing equalizing the pressure for Korhonen et al. [40] have proven the compartmental pressure to correlate well with regional biomechanical parameters.
Our second main finding was that oxygen consumption correlates with the biomechanical properties of the RF muscle, allowing describing up to 1/3 of the general VO2max with the elasticity of this muscle in studied triathletes. This is supported by the difference in elasticity of this muscle between the High and Low groups and in addition, the analysis between general decrement values from activated muscles with VO2max showed R=-0.45 with p=0.1. The main reason for the prominence of RF muscle is clearly the cycling and running events. Hein and Vain [41] showed that motion over the hip joint is related to the biomechanical properties of the agonist-antagonist muscles. They stated that the knee extension range of motion was primarily related to the elasticity of contracted posterior group of thigh muscles and that the tension (oscillation frequency) of m. semitendinosus was most responsible for limiting trunk forward flexion. This seems to support our finding that BF is stiffer in low endurance capable group, reflecting impeded locomotion over hip and/or knee joints when cycling or running. Zajac [42] explains, that the muscles producing highest work output during pedaling are uniarticular hip extensors (e.g. gluteus maximus) with anterior thigh muscles i.e. the uniarticular knee extensors (vastus medialis, lateralis, and intermedius) and that these muscles deliver their work output to both the crank and leg during leg extension. It has been shown that in cycling, the leg muscles exploit different metabolic paths than the ones of arms and that legs are responsible for using most of oxygen [43,44]. This knowledge seems to support our findings here. Cycling has been suggested to be crucial for successful running in triathlon [21,22]. Still, Hausswirth et al. [45] pointed out that a specific tactical decision, like drafting etc., allows triathletes to save considerable energy for running, suggesting also that this could mainly benefit good runners. Chapman et al. [46] and Candotti et al. [47] suggested that triathletes have a more robust cycling technique and that their recruitment of the BF muscle differs from cyclists. In transition from cycling to running, the recruitment of the tibialis anterior muscle seems not to be affected [27] and we did not see this muscle (neither the GC) to emerge. A recent study shows that in cyclists sprinting event, anterior thigh muscle plasticity accumulatively raised in a course of competition and measured leg flexors showed greater decrement values (lower elasticity/higher plasticity) than extensors. The 200m flying sprint—similarly to continuous maximum effort, affected most the elasticity of anterior thigh [48]. Differently from cyclists or triathletes, in endurance runners the VO2max is lower if it is measured on veloergometer compared to treadmill [49-54]. Therefore, it seems that running only does not allow fulfilling the cycling task with the same efficacy, while triathletes are able to run and cycle with similar intensity. In future, comparing triathletes with cyclists and runners may provide more knowledge about these issues.
Tissue elasticity (in a relaxed state) was strongly associated with both body weight and BMI in the way that more body mass was accompanied with higher plasticity. Negative correlation between the RF and BF muscle thickness (established by US) and decrement value was observed by Fröhlich-Zwahlen et al. [38] although they misinterpreted the results concerning the decrement value related to elasticity—the higher the decrement value, the less elastic or more plastic is the tissue. This means, that they observed higher elasticity at higher thickness in thigh muscles in non-athletes.
From previous research, it can be concluded that if a muscle is contracted, the force can be derived at least to some extent correctly from the stiffness or tension if measured alone (but not from elasticity; Jarocka et al. [29]). As these two parameters also depend on the stretch [28,55], the correlations between biomechanical parameters and the height allow us to suggest that in taller people muscles may be naturally more stretched and that fore stiffer. This may be the reflection of the effect of Gravity force on standing posture.
We also observed a trend between the VO2max and the elasticity in contracted BB (R= -0.47; p=0.09). Accompanied with the LD muscle showing better elasticity in a contracted state in the High group, these findings in these two muscles may reflect the effect of swimming trainings. On the other hand, as the contraction in our study was called out with a simple task, the excessive performance in the High group may have interfered causing these differences, for both muscles showed improved elasticity accompanied by slightly higher stiffness and tension [29]. It is a shortcoming of our work that the relaxed and contracted states were not registered by any other objective measures like EMG or generated force. It was our decision in order to simplify the procedure. As we calculated average over all the measurements, each single one less affects received values. Most recent study by Mencel et al. [56] cleared the doubts about accuracy of measurement point. According to their findings, the deviation up to 20% distally from muscle-belly is irrelevant in large muscles. Mencel et al. [56] used MyotonPRO, still the general principle of oscillatory measuring of the mentioned parameters is the same in both devices.
There are some aspects in our study that we want to emphasize. First, the study group is small. This may compromise the ability to translate our main finding to other sports. Important aspect is that all values are pooled over all three years. In each athlete, more than 30 measurement sessions were conducted. During this period, we observed that muscle parameters are strongly affected by a single training or competition event (data not published). Pooling of data abolishes these scattering effects. It is important to note that testing muscles only in a relaxed state definitely does not reveal all the valuable information and we recommend measuring also in the activated state. Contraction must be sufficient and standardized for each muscle, though.
Despite the rapidly growing number of publications on myotonometry, available material is scarce. Only a few aspects of these properties are covered in the literature, most of them describing stiffness or tension as properties and measurements made from tendons. Elasticity seems to be more stable and less volatile biomechanical property [57,58], that is also our experience, and authors are not reporting the results. That fore there is an urgent need for more studies using this methodology. According to most recent publication by Wdowski et al. [32], higher countermovement jump performance is enabled by higher elasticity (smaller decrement value), stiffness, and tone, with smaller creep-ability and quicker stress-relaxation times measured from Achilleus tendon. Two latter parameters are unique to newest myotonometric device [59-63].
In conclusion, myotonometry is a simple method to obtain objective information about the state and condition of muscles with the potential to monitor the training process. This would be beneficial in optimizing trainings, detecting muscle soreness in order to avoiding traumas, and monitoring rehabilitation. Large groups of athletes, separating sprinters and endurance athletes, in uniform events as running, cycling, and swimming are good candidates for future research.
Acknowledgements
The authors are grateful to Arved Vain, PhD, Dr. Habil. Biol., for his expertise; Prof. Vahur Ööpik, Dr Jüri Laasik, Kalle Karelson, Cand. of Biology, and Saima Timpmann, MSc, for their consultations and help. Thanks go to Jüri Käen, the coach of the triathletes; Inge Ringmets, MSc, and PhD student Kristiina Rajaleid for their help with the statistics and also to MD Adu Simisker for his expertise in ultrasonography.
Statements
This study was not funded by the interested parties, or by any other foundation.
All the identifiable information i.e. ages and other specific parameters are removed from the paper.
Conflict of Interest
- There is no duplicate publication elsewhere of any part of this work, there is no closely related paper published by us previously in this or in other journals.
- There is no conflict of interest of any sort between the authors and the study presented. This study was not funded by the interested parties, or by any other foundation.
- All authors were fully involved in the study or preparation of the manuscript. The typescript has been read and agreed by all authors.
- There are no competing interests bound to this study.
Author Contributions
GG contributed to conception and design of the study and made measurements on triathletes. AR participated in ultrasound (US) research. JT analysed the US video files with Matlab. IK and TM contributed to conception and design of the UH research and made the recordings. RV and EV contributed to data analysing and writings, RV additionally to US study concept. AV contributed to conception and design of the UH research, general data analysing, statistics and writing. All authors contributed to manuscript revision, read, and approved the submitted version.
References
- Grazi E. Water and muscle contraction. Int J Mol Sci 9 (2008): 1435-1452.
- Palmer BM, Wang Y, Miller MS. Distribution of myosin attachment times predicted from viscoelastic mechanics of striated muscle. J. Biomed. Biotechnol (2011): 592343.
- Williams CD, Regnier M, Daniel TL. Elastic energy storage and radial forces in the myofilament lattice depend on sarcomere length. PLoS Comp Biol 8 (2012).
- Kyröläinen H, Kivela R, Koskinen S, et al. Interrelationships between muscle structure muscle strength and running economy. Med Sci Sports Exerc 35 (2003): 45-49.
- Heyward VH. Advanced fitness assessment and exercise prescription. 3rd; Human Kinetics Champaign IL (1998).
- Sjödin B, Svedenhag J. Applied physiology of marathon running. Sports Med 2 (1985): 83-99.
- Fukashiro S, Abe T, Shibayama A, et al. Comparison of viscoelastic characteristics in triceps surae between Black and White athletes. Acta Physiol Scand 175 (2002): 183-187.
- Hanson P, Aagaard P, Magnusson SP. Biomechanical properties of isolated fascicles of the Iliopsoas and Achilles tendons in African American and Caucasian men. Ann Anat 194 (2012): 457-460.
- Wilson GJ, Murphy AJ, Pryor JF. Musculotendinous stiffness: its relationship to eccentric isometric and concentric performance. J Appl Physiol 76 (1994): 2714-2719.
- Kuitunen S, Komi PV, Kyröläinen H. Knee and ankle joint stiffness in sprint running. Med Sci Sports Exerc 34 (2002): 166-173.
- Rabita G, Couturier A, Lambertz D. Influence of training background on the relationships between plantarflexor intrinsic stiffness and overall musculoskeletal stiffness during hopping. Eur J Appl Physiol 103 (2008): 163-171.
- Slawinski J, Heubert R, Quievre J, et al. Changes in spring-mass model parameters and energy cost during track running to exhaustion. J Strength Cond Res 22 (2008): 930-936.
- Watsford M, Ditroilo M, Fernandez-Pena E, et al. Muscle stiffness and rate of torque development during sprint cycling. Med Sci Sports Exerc 42 (2010): 1324-1332.
- Dumke CL, Pfaffenroth CM, McBride JM, et al. Relationship between muscle strength power and stiffness and running economy in trained male runners. Int J Sports Physiol Perform 5 (2010): 249-261.
- Kim M-K, Kim C-S, Kim Y-W, et al. Mechanical Properties of Trunk Muscles using Myoton: Relationships with Physical Fitness of Trunk.: Medicine & Science in Sports and Exercise (2016).
- Hong JH, Dong-Hyeon Lee DH, Seong-Eun Kim SE, et al. Correlation between contraction ratio, endurance, and muscle tone of cervical muscles. Phys Ther Rehab Sci 9 (2020): 302-308.
- Berzosa C, Gutierrez H, Bascuas PJ, et al. Muscle Tone and Body Weight Predict Uphill Race Time in Amateur Trail Runners. Int. J. Environ. Res. Public Health 8 (2021): 2040.
- Jiménez-Sánchez C, Ortiz-Lucas M, Bravo-Esteban E, et al. Myotonometry as a measure to detect myofascial trigger points: an inter-rater reliability study. Physiol Meas 39 (2018): 115004.
- Knechtle B, Kohler G. Running performance not anthropometric factors is associated with race success in a Triple Iron Triathlon. Br J Sports Med 43 (2009): 437-441.
- Sleivert GG, Rowlands DS. Physical and physiological factors associated with success in the triathlon. Sports Med 22 (1996): 8-18.
- Bonacci J, Green D, Saunders PU, et al. Plyometric training as an intervention to correct altered neuromotor control during running after cycling in triathletes: a preliminary randomised controlled trial. Phys Ther Sport 12 (2011): 15-21.
- Bonacci J, Saunders PU, Alexander M, et al. Neuromuscular control and running economy is preserved in elite international triathletes after cycling. Sports Biomech 10 (2011): 59-71.
- Cavagna GA, Komarek L, Mazzoleni S. The mechanics of sprint running. J Physiol 217 (1971): 709-721.
- Kram R, Dawson TJ. Energetics and biomechanics of locomotion by red kangaroos (Macropus rufus). Comp Biochem Physiol B Biochem Mol Biol 120 (1998): 41-49.
- Muthupillai R, Lomas DJ, Rossman PJ, et al. Magnetic resonance elastography by direct visualization of propagating acoustic strain waves. Science 269 (1995): 1854-1857.
- Gennisson JL, Cornu C, Catheline S, et al. Human muscle hardness assessment during incremental isometric contraction using transient elastography. J Biomech 38 (2005): 1543-1550.
- Eby SF, Song P, Chen S, et al. Validation of shear wave elastography in skeletal muscle. J Biomech 46 (2013): 2381-2387.
- Ditroilo M, Hunter AM, Haslam S, et al. The effectiveness of two novel techniques in establishing the mechanical and contractile responses of biceps femoris. Physiol Meas 32 (2011): 1315-1326.
- Jarocka E, Marusiak J, Kumorek M, et al. Muscle stiffness at different force levels measured with two myotonometric devices. Physiol Meas 33 (2011): 65-78.
- Gervasi M, Sisti D, Amatori S, et al. Muscular viscoelastic characteristics of athletes participating in the European Master Indoor Athletics Championship. Eur J Appl Physiol 117 (2017): 1739-1746.
- Pozarowszczyk B, Pawlaczyk W, Smoter M, et al. Effects of Karate Fights on Achilles Tendon Stiffness Measured by Myotonometry. J Hum Kinet 56 (2017): 93-97.
- Wdowski MM, Rosicka K, Hill MW. Influence of lower-limb muscular and tendon mechanical properties and strength on countermovement jump performance. J Sports Med Phys Fitness 63 (2023): 16-23.
- Bizzini M, Mannion AF. Reliability of a new hand-held device for assessing skeletal muscle stiffness. Clin Biomech. (Bristol Avon) 18 (2003): 459-461.
- Zinder SM, Padua DA. Reliability validity and precision of a handheld myometer for assessing in vivo muscle stiffness. J Sport Rehab. Technical Notes . 20(2011): 2010-0051.
- Kelly JP, Koppenhaver SL, Michener LA, et al. Characterization of tissue stiffness of the infraspinatus, erector spinae, and gastrocnemius muscle using ultrasound shear wave elastography and superficial mechanical deformation. J Electromyogr Kinesiol 38 (2018): 73-80.
- Li YP, Liu CL, Zhang ZJ. Feasibility of Using a Portable MyotonPRO Device to Quantify the Elastic Properties of Skeletal Muscle. Med Sci Monit 28 (2022): e934121.
- Bravo-Sánchez A, Abián P, Sánchez-Infante J, et al. Objective Assessment of Regional Stiffness in Vastus Lateralis with Different Measurement Methods: A Reliability Study. Sensors (Basel) 21 (2021): 3213.
- Fröhlich-Zwahlen AK, Casartelli NC, Item-Glatthorn JF, et al. Validity of resting myotonometric assessment of lower extremity muscles in chronic stroke patients with limited hypertonia: a preliminary study. J Electromyogr Kinesiol 24 (2014): 762-769.
- Gavronski G, Veraksits A, Vasar E, et al. Evaluation of viscoelastic parameters of the skeletal muscles in junior triathletes. Physiol Meas 28 (2007): 625-637.
- Korhonen RK, Vain A, Vanninen E, et al. Can mechanical myotonometry or electromyography be used for the prediction of intramuscular pressure? Physiol Meas 26 (2005): 951-963.
- Hein V, Vain A. Joint mobility and the oscillation characteristics of muscle. Scand J Med Sci Sports 8 (1998): 7-13.
- Zajaca FE. Borelli Lecture from the American Society of Biomechanics Conference 2001: Understanding muscle coordination of the human leg with dynamical simulations. J Biomech 35 (2002): 1011-1018.
- Volianitis S, Secher NH. Arm blood flow and metabolism during arm and combined arm and leg exercise in humans. J Physiol 544 (2002): 977-984.
- Calbet JA, Gonzalez-Alonso J, Helge JW, Søndergaard H, Munch-Andersen T, Boushel R and Saltin B. Cardiac output and leg and arm blood flow during incremental exercise to exhaustion on the cycle ergometer. J Appl Physiol 103 (2007): 969-978.
- Hausswirth C, Lehenaff D, Dreano P, et al. Effects of cycling alone or in a sheltered position on subsequent running performance during a triathlon. Med Sci Sports Exerc 31 (1999): 599-604.
- Chapman AR, Vicenzino B, Blanch P, et al. Does cycling effect motor coordination of the leg during running in elite triathletes? J Sci Med Sport 11 (2008): 371-380.
- Candotti CT, Loss JF, Bagatini D, et al. Cocontraction and economy of triathletes and cyclists at different cadences during cycling motion. J Electromyogr Kinesiol 19 (2009): 915-921.
- Klich S, Krymski I, Kawczynski A. Viscoelastic properties of lower extremity muscles after elite track cycling sprint events: A case report. Cent Eur J Sport Sci Med 1 (2020): 5-10.
- Kohrt WM, O'Connor JS, Skinner JS. Longitudinal assessment of responses by triathletes to swimming cycling and running. Med Sci Sports Exerc 21 (1989): 569-575.
- Hue O, Le Gallais D, Boussana A, et al. Performance level and cardiopulmonary responses during a cycle-run trial. Int J Sports Med 21 (2000): 250-255.
- Basset FA, Boulay MR. Treadmill and cycle ergometer tests are interchangeable to monitor triathletes annual training. J Sports Sci Med 2 (2003): 110-116.
- Davis JA, Tyminski TA, Soriano AC, et al. Exercise test mode dependency for ventilatory efficiency in women but not men. Clin Physiol Funct Imaging 26 (2006): 72-78.
- Millet GP, Vleck VE, Bentley DJ. Physiological differences between cycling and running: lessons from triathletes Sports Med 39 (2009): 179-206.
- Carey DG, Tofte C, Pliego GJ, et al. Transferability of running and cycling training zones in triathletes: implications for steady-state exercise. J Strength Cond Res 23 (2009): 251-258.
- Huang J, Qin K, Tang C, et al. Assessment of Passive Stiffness of Medial and Lateral Heads of Gastrocnemius Muscle, Achilles Tendon, and Plantar Fascia at Different Ankle and Knee Positions Using the MyotonPRO. Med Sci Monit 24 (2018): 7570-7576.
- Mencel J, Marusiak J, Jaskólska A, et al. Impact of the Location of Myometric Measurement Points on Skeletal Muscle Mechanical Properties Outcomes. Muscle Ligament and Tendon Journal 11 (2021): 525-535.
- Vain A, Kums T, Ereline J, et al. Gastrocnemius muscle tone, elasticity, and stiffness in association with postural control characteristics in young men. Proc Est Acad Sci 64 (2015): 525-534.
- Julià-Sánchez S, Álvarez-Herms J, Cirer-Sastre R, et al. The Influence of Dental Occlusion on Dynamic Balance and Muscular Tone. Front Physiol 10 (2020): 1626.
- Agyapong-Badu S, Warner M, Samuel D, et al. Measurement of ageing effects on muscle tone and mechanical properties of rectus femoris and biceps brachii in healthy males and females using a novel hand-held myometric device. Arch Gerontol Geriatr (2015).
- Chapman AR, Vicenzino B, Blanch P, et al. Leg muscle recruitment during cycling is less developed in triathletes than cyclists despite matched cycling training loads. Exp Brain Res 181 (2007): 503-518.
- Gapeyeva H, Vain A, Pääsuke M, et al. A tone of skeletal muscles and skin fold thickness in 15 to 17-year-old girls. Proceedings of the International Conference "Movement and Health in the Value System of People at the Beginning of the Millennium". Nitra (2000): 266-271.
- Pruyn EC, Watsford ML, Murphy AJ. Validity and reliability of three methods of stiffness assessment. J Sport Health Sci 5 (2016): 476-483.
- Pozarowszczyk B, Pawlaczyk W, Smoter M, et al. Effects of Karate Fights on Achilles Tendon Stiffness Measured by Myotonometry. J Hum Kinet 56 (2017): 93-97.