Liquiritigenin Enhances the Inhibitory Effects of the Cholesterol Biosynthesis Inhibitor RO 48-8071 on Cell Viability in Ovarian-Cancer Cells in Vitro
Article Information
Yayun Liang and Salman M Hyder*
Dalton Cardiovascular Research Center and Department of Biomedical Sciences University of Missouri, Columbia 65211, United States
*Corresponding Author: Dr. Salman M. Hyder, Dalton Cardiovascular Research Center, University of Missouri-Columbia, 134 Research Park Drive, Columbia, MO 65203, United States.
Received: 14 August 2023; Accepted: 30 August 2023; Published: 20 September 2023
Citation:
Yayun Liang, Salman M Hyder. Liquiritigenin Enhances the Inhibitory Effects of the Cholesterol Biosynthesis Inhibitor RO 48-8071 on Cell Viability in Ovarian-Cancer Cells in Vitro. Journal of Cancer Science and Clinical Therapeutics. 7 (2023): 158-163.
View / Download Pdf Share at FacebookAbstract
Almost 25,000 new cases of epithelial ovarian cancer (EOC) are reported each year in the United States. Cancers of the ovary have poor prognosis due to drug resistance and metastasis, and have the highest mortality rate of all the known gynecological malignancies. Despite concerted efforts to develop new strategies for preventing and treating ovarian cancer, novel and more effective non-toxic therapies for ovarian cancer are urgently needed. Recent observations show that RO 48-8071 ([4’-[6-(Allylmethylamino)hexyloxy]-4-bromo-2’-fluorobenzophenone fumarate] [RO], a small-molecule inhibitor of the key cholesterol biosynthesis enzyme 2, 3-oxidosqualene cyclase, inhibits breast and prostate cancer cells. RO also induces the tumor-suppressor protein estrogen receptor (ER) β in both breast- and prostate-cancer cells, and also inhibits growth of ovarian-cancer cells both in vitro and in vivo. Extending upon these earlier studies, here we found that RO also induces ERβ expression in OVCAR-3 ovarian-cancer cells. Further, we demonstrated that treatment of two ovarian-cancer cell lines (OVCAR-3 and SK-OV-3) with liquiritigenin (LQ), a naturally occurring compound that is an ERβ agonist, reduced cell viability in vitro. When we treated OVCAR-3 and SK-OV-3 cells with RO, LQ, or RO + LQ, we observed that RO + LQ combination treatment synergistically reduced cell viability. Further in vivo studies examining the effects of RO + LQ combination treatment on EOC are warranted, as a means of exploring a potential new therapeutic approach to combat ovarian cancers with minimal toxicity.
Keywords
Ovarian cancer; Estrogen receptor-β; RO 48-8071; Liquiritigenin
Ovarian cancer articles; Estrogen receptor-β articles; RO 48-8071 articles; Liquiritigenin articles
Ovarian cancer articles Ovarian cancer Research articles Ovarian cancer review articles Ovarian cancer PubMed articles Ovarian cancer PubMed Central articles Ovarian cancer 2023 articles Ovarian cancer 2024 articles Ovarian cancer Scopus articles Ovarian cancer impact factor journals Ovarian cancer Scopus journals Ovarian cancer PubMed journals Ovarian cancer medical journals Ovarian cancer free journals Ovarian cancer best journals Ovarian cancer top journals Ovarian cancer free medical journals Ovarian cancer famous journals Ovarian cancer Google Scholar indexed journals Estrogen receptor-β articles Estrogen receptor-β Research articles Estrogen receptor-β review articles Estrogen receptor-β PubMed articles Estrogen receptor-β PubMed Central articles Estrogen receptor-β 2023 articles Estrogen receptor-β 2024 articles Estrogen receptor-β Scopus articles Estrogen receptor-β impact factor journals Estrogen receptor-β Scopus journals Estrogen receptor-β PubMed journals Estrogen receptor-β medical journals Estrogen receptor-β free journals Estrogen receptor-β best journals Estrogen receptor-β top journals Estrogen receptor-β free medical journals Estrogen receptor-β famous journals Estrogen receptor-β Google Scholar indexed journals RO 48-8071 articles RO 48-8071 Research articles RO 48-8071 review articles RO 48-8071 PubMed articles RO 48-8071 PubMed Central articles RO 48-8071 2023 articles RO 48-8071 2024 articles RO 48-8071 Scopus articles RO 48-8071 impact factor journals RO 48-8071 Scopus journals RO 48-8071 PubMed journals RO 48-8071 medical journals RO 48-8071 free journals RO 48-8071 best journals RO 48-8071 top journals RO 48-8071 free medical journals RO 48-8071 famous journals RO 48-8071 Google Scholar indexed journals Liquiritigenin articles Liquiritigenin Research articles Liquiritigenin review articles Liquiritigenin PubMed articles Liquiritigenin PubMed Central articles Liquiritigenin 2023 articles Liquiritigenin 2024 articles Liquiritigenin Scopus articles Liquiritigenin impact factor journals Liquiritigenin Scopus journals Liquiritigenin PubMed journals Liquiritigenin medical journals Liquiritigenin free journals Liquiritigenin best journals Liquiritigenin top journals Liquiritigenin free medical journals Liquiritigenin famous journals Liquiritigenin Google Scholar indexed journals Fetal Bovine Serum articles Fetal Bovine Serum Research articles Fetal Bovine Serum review articles Fetal Bovine Serum PubMed articles Fetal Bovine Serum PubMed Central articles Fetal Bovine Serum 2023 articles Fetal Bovine Serum 2024 articles Fetal Bovine Serum Scopus articles Fetal Bovine Serum impact factor journals Fetal Bovine Serum Scopus journals Fetal Bovine Serum PubMed journals Fetal Bovine Serum medical journals Fetal Bovine Serum free journals Fetal Bovine Serum best journals Fetal Bovine Serum top journals Fetal Bovine Serum free medical journals Fetal Bovine Serum famous journals Fetal Bovine Serum Google Scholar indexed journals 3-oxidosqualene cyclase articles 3-oxidosqualene cyclase Research articles 3-oxidosqualene cyclase review articles 3-oxidosqualene cyclase PubMed articles 3-oxidosqualene cyclase PubMed Central articles 3-oxidosqualene cyclase 2023 articles 3-oxidosqualene cyclase 2024 articles 3-oxidosqualene cyclase Scopus articles 3-oxidosqualene cyclase impact factor journals 3-oxidosqualene cyclase Scopus journals 3-oxidosqualene cyclase PubMed journals 3-oxidosqualene cyclase medical journals 3-oxidosqualene cyclase free journals 3-oxidosqualene cyclase best journals 3-oxidosqualene cyclase top journals 3-oxidosqualene cyclase free medical journals 3-oxidosqualene cyclase famous journals 3-oxidosqualene cyclase Google Scholar indexed journals tumor cells articles tumor cells Research articles tumor cells review articles tumor cells PubMed articles tumor cells PubMed Central articles tumor cells 2023 articles tumor cells 2024 articles tumor cells Scopus articles tumor cells impact factor journals tumor cells Scopus journals tumor cells PubMed journals tumor cells medical journals tumor cells free journals tumor cells best journals tumor cells top journals tumor cells free medical journals tumor cells famous journals tumor cells Google Scholar indexed journals multifocal intraperitoneal dissemination articles multifocal intraperitoneal dissemination Research articles multifocal intraperitoneal dissemination review articles multifocal intraperitoneal dissemination PubMed articles multifocal intraperitoneal dissemination PubMed Central articles multifocal intraperitoneal dissemination 2023 articles multifocal intraperitoneal dissemination 2024 articles multifocal intraperitoneal dissemination Scopus articles multifocal intraperitoneal dissemination impact factor journals multifocal intraperitoneal dissemination Scopus journals multifocal intraperitoneal dissemination PubMed journals multifocal intraperitoneal dissemination medical journals multifocal intraperitoneal dissemination free journals multifocal intraperitoneal dissemination best journals multifocal intraperitoneal dissemination top journals multifocal intraperitoneal dissemination free medical journals multifocal intraperitoneal dissemination famous journals multifocal intraperitoneal dissemination Google Scholar indexed journals gynecological malignancies articles gynecological malignancies Research articles gynecological malignancies review articles gynecological malignancies PubMed articles gynecological malignancies PubMed Central articles gynecological malignancies 2023 articles gynecological malignancies 2024 articles gynecological malignancies Scopus articles gynecological malignancies impact factor journals gynecological malignancies Scopus journals gynecological malignancies PubMed journals gynecological malignancies medical journals gynecological malignancies free journals gynecological malignancies best journals gynecological malignancies top journals gynecological malignancies free medical journals gynecological malignancies famous journals gynecological malignancies Google Scholar indexed journals toxic chemotherapeutic drugs articles toxic chemotherapeutic drugs Research articles toxic chemotherapeutic drugs review articles toxic chemotherapeutic drugs PubMed articles toxic chemotherapeutic drugs PubMed Central articles toxic chemotherapeutic drugs 2023 articles toxic chemotherapeutic drugs 2024 articles toxic chemotherapeutic drugs Scopus articles toxic chemotherapeutic drugs impact factor journals toxic chemotherapeutic drugs Scopus journals toxic chemotherapeutic drugs PubMed journals toxic chemotherapeutic drugs medical journals toxic chemotherapeutic drugs free journals toxic chemotherapeutic drugs best journals toxic chemotherapeutic drugs top journals toxic chemotherapeutic drugs free medical journals toxic chemotherapeutic drugs famous journals toxic chemotherapeutic drugs Google Scholar indexed journals
Article Details
Abbreviations:
ANOVA: one-way analysis of variance; EOC: Epithelial Ovarian Cancer; ER: Estrogen Receptor; FBS: Fetal Bovine Serum; LQ: Liquiritigenin; OSC: 2, 3-oxidosqualene cyclase; PBS: Phosphate-Buffered Saline; RO: RO 48-8071 ([4’-[6-(Allylmethylamino)hexyloxy]-4-bromo-2’-fluorobenzophenone fumarate]); SEM: Standard Error of the Mean; SRB: Sulforhodamine B
1. Introduction
Almost 25,000 new cases of epithelial ovarian cancer (EOC) are diagnosed annually in the United States [1]. EOC is a deadly disease characterized by aggressively growing tumor cells and often results in multifocal intraperitoneal dissemination, accompanied by intense neovascularization [2]. Because EOC tumors are often detected at a late stage, they are generally non-responsive to chemotherapy, have poor prognosis, and demonstrate the highest mortality rate of all the known gynecological malignancies [3]. Standard treatments include systemic high-dose, toxic chemotherapeutic drugs; however, drug resistance almost always occurs, and cancers originating in the ovary often metastasize to distant organs [3,4]. More than half of ovarian-cancer patients die from this resulting metastasis; thus, the outlook for those afflicted with the disease is generally bleak [5]. For these reasons, efforts to develop new strategies for preventing and treating ovarian cancer have been undertaken. Although novel and more effective non-toxic therapies for ovarian cancer are urgently needed, thus far success in this regard has been limited.
Cholesterol is an essential structural and functional component of cellular membranes and cellular metabolism, and is therefore required for tumor growth [6,7]. Endogenous steroid hormones, including estradiol, are derived from the metabolic precursor cholesterol, which itself can be modified to 27-hydroxy cholesterol. 27-hydroxy cholesterol can serve as a selective estrogen-receptor modulator that increases estrogen signaling in some tissues, including in a subset of ovarian-cancer cells. Because ovarian-cancer cells express enzymes involved in the biosynthesis of endogenous cholesterol [8,9], inhibition of cholesterol synthesis may be an effective means by which to arrest tumor-cell proliferation; furthermore, the combined use of cholesterol biosynthesis inhibitors and commonly used chemotherapeutic drugs may provide a novel and hitherto untested approach to combatting the progression of ovarian cancer. Statins have been used to inhibit HMG-CoA reductase, an enzyme that is essential for cholesterol biosynthesis, but statin therapy could cause some undesirable side effects, attributed to reduced levels of isoprenoids, defective post-translational modification of membrane proteins, and impaired membrane structure and function [10]. Thus, alternative approaches to inhibit cholesterol biosynthesis are being considered. 2, 3-oxidosqualene cyclase (OSC), which converts 2, 3-monoepoxysqualene to lanosterol, a key step in the biosynthesis of cholesterol, has recently emerged as a possible new target for inhibiting the cholesterol-biosynthetic pathway [11]. Because OSC functions downstream of HMG-CoA reductase during cholesterol biosynthesis, it is not likely to be associated with the same adverse effects as statins. Potent small-molecule inhibitors of OSC, such as RO 48-8071 ([4’-[6-(Allylmethylamino)hexyloxy]-4-bromo-2’-fluorobenzophenone fumarate]; RO) have been identified [11-14].
We recently showed that RO inhibits growth of ovarian-cancer cells, both in vitro and in vivo (13). RO also inhibits the growth of other types of cancer, and, in some of these (including breast and prostate cancer), RO induces estrogen receptor-b (ERb), which acts as a tumor-suppressor protein in most cancers [15-18]. In this study, we tested whether RO could also induce ERb in EOC in vitro, and whether liquiritigenin (LQ), a natural non-toxic plant product and an agonist of ERb [19-21], could enhance the inhibitory effects of RO on cell viability in ovarian-cancer cells, as it does in breast-cancer cells [15,16].
2. Materials and Methods
2.1 Reagents
Antibody to detect ERb was obtained from Santa Cruz Biotechnology (Dallas, TX) (H-150). The b-Actin antibody clone (AC-74), was obtained from Sigma (St Louis, MO). Sulforhodamine B (SRB) was obtained from Sigma (St. Louis, MO). BD Matrigel Matrix was purchased from BD Biosciences (Bedford, MA). RO and LQ were bought from Tocris (Bristol, UK).
2.2 Cell lines and cell culture
Epithelial ovarian cancer cell lines OVCAR-3 (high grade serous) and SK-OV-3 (non-high grade serous) were obtained from ATCC (Manassas, VA, USA). Cell-culture media, phosphate-buffered saline (PBS), and 0.05% trypsin-EDTA were purchased from Invitrogen Corporation & Life Technologies (Grand Island, NY). Fetal bovine serum (FBS) was bought from JRH Biosciences (Lenexa, KS). OVCAR-3 cells were maintained in 20% FBS RPMI-1640, while SK-OV-3 cells were maintained in 10% FBS McCoy’s 5a medium. All cells were grown in 100 × 20 mm tissue-culture dishes and harvested with 0.05% trypsin-EDTA. During treatment of cells with RO or LQ, the percent FBS was reduced by half for the duration of treatment so that the process of reduction of cell viability and/or apoptosis would not be rescued with serum.
2.3 Cell-viability assay
An SRB assay was used to measure cell viability, as previously described [12-14]. Briefly, cells were grown to 70% confluence, then, following trypsin treatment, cells were seeded into 96-well plates in 100 ml growth medium and incubated overnight at 37ºC with 5% CO2. Cells were washed once with media and incubated for 24 or 48 h in 100 ml medium with 10% FBS (OVCAR-3 cells) or 5% FBS (SK-OV-3 cells) in their respective media, in the presence of RO (0–10 mM), LQ (0–600 mM), or RO (0–10 mM) + LQ (0–300 mM). At the end of the incubation, surviving or adherent cells were fixed in situ by adding 100 ml 50% cold trichloroacetic acid for 1 h at 4ºC. Cells were washed with ice water, dried, and stained with 50 ml 4% SRB for 8 min at room temperature. Unbound stain was removed by washing cells 5X with cold 1% acetic acid, then the cells were dried at room temperature. Cell-bound stain was solubilized in 150 ml 10 mM Tris buffer and quantified at 520 nm using a SpecTRA MAX 190 microplate reader (Sunnyvale, CA). Six wells were used for each dose/experimental condition and each experiment was performed at least twice.
2.4 Western blots
Whole-cell extracts were prepared with a nuclear extraction TransAm kit (Active Motif, Carlsbad, CA) and Western blotting performed as previously described [15,16].
2.5 Statistical analysis
Statistical significance was measured using one-way analysis of variance (ANOVA) with repeated measure over time. When necessary, it was assumed that ANOVA was non-parametric. Values are reported as mean ± standard error of the mean (SEM). For samples with significant F-ratio (p<0.05), the Student-Newman-Keuls multirange test was employed (SigmaStat).
3. Results
Because the cholesterol-biosynthesis inhibitor RO has been shown to induce the anti-proliferative protein ERb in both breast- and prostate-cancer cells and to reduce their viability [15,16], we conducted studies to ascertain whether exposure to RO could also induce ERb in ovarian-cancer cells. Using OVCAR-3, an aggressive ovarian-cancer cell line, we found that ERb expression was induced by both 10 mM and 25 mM RO (Figure 1). In order to determine whether an ERb agonist will enhance the anti-proliferrative effect of RO, we tested LQ, a natural ligand that serves as an agoinist of ERb selectively [17,18]. When we examined the ability of high doses of the ERb agonist LQ to inhibit the viability of ovarian-cancer cells, we found that 24-h or 48-h exposure to LQ reduced the viability of both OVCAR-3 and SK-OV-3 ovarian-cancer cells (Figure 2). The IC50 values for OVCAR-3 cells and SK-OV-3 cells were 296 + 7 mM and 501 + 13 mM respectively, in a 48-h SRB assay, demonstrating that SK-OV-3 cells were less sensitive than OVCAR-3 cells to LQ treatment.

Figure 1: RO increases ERb expression in OVCAR-3 ovarian-cancer cells.
OVCAR-3 cells were treated with the indicated concentrations of RO in 10% FBS RPMI-1640 medium for 7 h. Control cells (C) were treated with vehicle (10% FBS RPMI-1640 + PBS). Cell extracts were prepared, and 50 mg protein/sample was loaded onto 10% NuPAGE Bis-Tris gels (Invitrogen, Carlsbad, CA), electrophoresed, then transferred to PVDF membrane (BioRad, Hercules, CA). Membranes were probed with anti-ERb antibody (1:200 dilution), followed by anti-rabbit secondary antibody (1:3000 dilution). Immunoreactive bands were visualized using the ECL Plus detection kit (Amersham, Arlington Heights, IL). Beta-Actin expression was probed on same blots for demonstrating equal loading of protein. The experiment was performed twice.
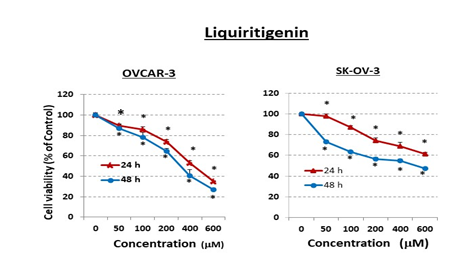
Figure 2: Liquiritigenin (LQ) reduces ovarian-cancer cell viability in vitro.
Ovarian-cancer cells (OVCAR-3: 6 × 103/well and SK-OV-3: 5 × 103/well) were seeded into 96-well plates overnight. The next day, cells were washed and treated with pharmacological doses of LQ (mM) for 24 or 48 h in 10% FBS RPMI-1640 medium (OVCAR-3) or in 5% FBS McCoy’s 5a medium (SK-OV-3). Control cells (0 mM) were incubated with vehicle (same medium + PBS). Cell viability was determined by SRB assay. Values represent means ± SEM (n=6). * Significant difference compared with control group (set at 100%) (p<0.05 using ANOVA).
Given our finding here that RO induces ERb in ovarian-cancer cells, and our previous findings that RO reduces cell viability of breast-cancer cells to some extent at 5 and 10 mM in an in vitro assay [13-15], we sought to determine whether LQ could enhance the effects of RO and lower the concentrations of RO needed to reduce cell viability in ovarian-cancer cells. To examine this possibility, we examined the viability of OVCAR-3 and SK-OV-3 cells exposed to 5 or 10 mM RO together with 100, 200, or 300 mM LQ. Both cell lines were also incubated with RO or LQ alone. In all cases, cells were pre-treated with either 5 or 10 mM RO for 6-7 h to increase the levels of ERb (as shown in Figure 1). Exposure of OVCAR-3 cells to either RO (5 or 10 mM) or LQ (100–300 mM) alone resulted in a modest reduction of cell viability (Figure 3). However, RO + LQ combination treatment reduced cell viability synergistically in a 20-h assay. For example, OVCAR-3 viability was reduced by approximately 10% in response to exposure to either 5 mM RO or 100 mM LQ alone, while a combination of RO + LQ at these concentrations reduced viability by approximately 40%.
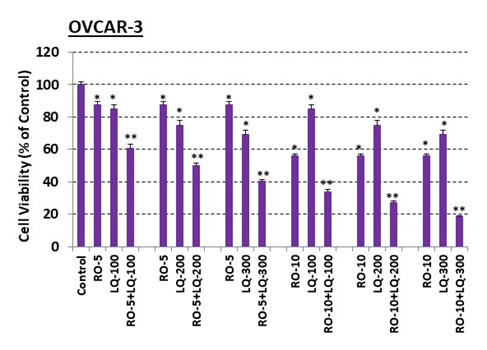
Figure 3: Liquiritigenin (LQ) enhances RO effects on OVCAR-3 ovarian-cancer cell viability.
OVCAR-3 cells (5 × 103/well) were seeded into 96-well plates overnight. The next day, cells were washed and treated with 5 mM or 10 mM RO for 7 h. Cells were then washed and treated with 5 mM or 10 mM RO, 100, 200, or 300 mM LQ, or 5 or 10 mM RO in combination with 100, 200, or 300 mM LQ for another 20 h in 10% FBS RPMI-1640 medium. Control cells were incubated with vehicle (same medium + PBS). Cell viability was determined by SRB assay. Values represent means ± SEM (n=6). * Significant difference compared with control group (set at 100%) (p<0.05 using ANOVA). ** Significant difference compared with control, RO alone, and LQ alone groups (p<0.05 using ANOVA).

Figure 4: Liquiritigenin (LQ) enhances RO effects on SK-OV-3 ovarian-cancer cell viability.
SK-OV-3 cells (4 x 103/well) were seeded into 96-well plates overnight. The next day, cells were washed and treated with 5 mM or 10 mM RO for 6 h. Cells were then washed and treated with 5 mM or 10 mM RO, 100, 200, or 300 mM LQ, or 5 or 10 mM RO in combination with 100, 200, or 300 mM LQ for another 18 h (A) or 44 h (B) in 5% FBS McCoy’s 5a medium. Control cells were incubated with vehicle (same medium + PBS). Cell viability was determined by SRB assay. Values represent means ± SEM (n=6). * Significant difference compared with control group (set at 100%) (p<0.05 using ANOVA). ** Significant difference compared with control, RO alone, and LQ alone groups (p<0.05 using ANOVA).
When we conducted this same analysis in SK-OV-3 cells, as we observed with OVCAR-3 cells, viability was reduced by RO alone, while RO + LQ acted synergistically to reduce cell viability in the short-term (18 h) assay (Figure 4A). To determine whether exposure of ovarian-cancer cells to RO and LQ for prolonged periods might cause an even greater effect on viability, we exposed SK-OV-3 cells to the same concentrations of the two compounds used in the studies presented in Figure 4A, but for a longer time (44 h). Under these conditions, a combination of a higher concentration of RO (10 mM) and 100–300 mM LQ resulted in an approximately 90–95% loss of cell viability, while cells subjected to single-agent treatment exhibited viability reduced by approximately 70% and 40%, respectively, with RO and LQ alone (Figure 4B).
4. Discussion
Extending on our previous reports that the cholesterol biosynthesis inhibitor RO induces ERb in prostate- and breast-cancer cells [15,16], here we show that RO also induces ERb in ovarian-cancer cells in vitro. Although the mechanism by which this ERb induction occurs within cancer cells remains unclear, the potential therapeutic significance of this finding is enormous, given that ERb is generally regarded as a tumor suppressor in most cell types [17-19]. Our studies reported here also show that, in accord with observations in a variety of cancer-cell lines, pharmacological levels of LQ effectively reduced the viability of OVCAR-3 and SK-OV-3 ovarian-cancer cells, both of which are difficult to treat with current drug regimens. However, the reduction in viability observed for both of these cell lines increased dramatically when the cells were exposed to a combination of LQ and the ERb inducer RO. For this reason, RO + LQ combination therapies may represent an attractive option for treating patients with ovarian cancer.
ERb has previously been shown to reduce the effective dose of certain drugs, though the exact mechanism for such an effect is not known at present [22]. For example, it can be envisioned that ERb not only induces a different complement of genes than its proliferative counterpart ERa, but that it may also inhibit the proliferative signal-transduction pathways, although this possibility needs to be fully investigated. Further, ERb has also been shown to inhibit cholesterol biosynthesis [23,24], and thus could synergize with RO in this manner. If utilized, combination RO + LQ treatments could effectively inhibit ovarian-cancer progression and might also reduce the need for potentially toxic chemotherapeutic regimens such as those currently employed, increasing the quality of life of those suffering from this disease. Although our observations regarding the effectiveness of RO + LQ are based on cell-culture studies, we expect that the effects we observed in vitro will be translatable to in vivo models, given that we have already demonstrated that RO on its own quite effectively inhibits the in vivo growth of EOC xenografts in an animal model [13].
Treatment of ovarian cancer with anti-cancer drugs such as cisplatin is somewhat successful in arresting tumor progression, but is accompanied by extreme side effects and the development of drug resistance [25]. Our research explored the effectiveness of modulating estrogen signaling due to induction of ERb by the OSC inhibitor RO in ovarian-cancer cells. We demonstrated that treatment of ovarian-cancer cells with RO increased their sensitivity to the natural ERb agonist LQ, which binds to ERb, thereby reducing their viability. Based on our observations, we propose that low-dose combinations of RO and LQ should be further explored as a potential new therapy for combatting ovarian cancer. Our findings contribute to ongoing efforts to identify novel, effective treatments for primary and metastatic ovarian cancer that employ new non-toxic drugs.
Acknowledgements
This research was supported by a Faculty COR award from the College of Veterinary Medicine, University of Missouri-Columbia. SMH is the Zalk Missouri Professor of Tumor Angiogenesis.
References
- Siegel RL, Miller KD, Jemal A. Cancer statistics. CA Cancer J Clin 70 (2020): 7-30.
- Momenimovahed Z, Tiznobaik A, Taheri S, et al. Ovarian cancer in the world: epidemiology and risk factors. Int J Womens Health 11 (2019): 287-299.
- Yeung TL, Leung CS, Yip KP, et al. Cellular and molecular processes in ovarian cancer metastasis. A Review in the Theme: Cell and Molecular Processes in Cancer Metastasis. Am J Physiol Cell Physiol 309 (2015): C444-C456.
- Vaughan S, Coward JI, Bast RC Jr, et al. Rethinking ovarian cancer: recommendations for improving outcomes. Nat Rev Cancer 11 (2011): 719-725.
- Yousefi M, Dehghani S, Nosrati R, et al. Current insights into the metastasis of epithelial ovarian cancer - hopes and hurdles. Cell Oncol 43 (2020): 515-538.
- Kuzu OF, Noory MA, Robertson GP. The Role of Cholesterol in Cancer. Cancer Res 76 (2016): 2063-2070.
- Giacomini I, Gianfanti F, Desbats MA, et al. Cholesterol Metabolic Reprogramming in Cancer and Its harmacological Modulation as Therapeutic Strategy. Front Oncol 11 (2021): 682911.
- Yang J, Wang L, Jia R. Role of de novo cholesterol Synthesis. J Cancer 11 (2020): 1761-1767.
- He J, Siu MKY, Ngan HYS, et al. (2021) Aberrant Cholesterol Metabolism in Ovarian Cancer: Identification of Novel Therapeutic Targets. Front Oncol 11: 738177.
- Liao JK. Isoprenoids as mediators of the biological effects of statins. J Clin Invest 110 (2002): 285-288.
- Thoma R, Schulz-Gasch T, D'Arcy B, et al. Insight intosteroid scaffold formation from the structure of humanoxidosqualene cyclase. Nature 432 (2004): 118-122.
- Grinter SZ, Liang Y, Huang S-Y, et al. An inverse docking approach for identifying new potential anti-cancer targets. J. Molecular Graphics and Modelling 29 (2011): 795-799.
- Liang Y, Nephew KP, Hyder SM. Cholesterol Biosynthesis Inhibitor RO 48-8071 Suppresses Growth of Epithelial Ovarian Cancer Cells in Vitro and In Vivo. Journal of Cancer Science and Clinical Therapeutics 7 (2023): 1-8.
- Liang Y, Zou X, Hyder SM. Cholesterol biosynthesis inhibitor RO 48-8071 inhibits viability of aggressive cancer cells. Cancer Rep Rev 4 (2020): 1-4.
- Liang Y, Besch-Williford C, Aebi JD, et al. Cholesterol biosynthesis inhibitors as potent novel anti-cancer agents: suppression of hormone-dependent breast cancer by the oxidosqualene cyclase inhibitor RO 48-8071. Breast Cancer Res Treat 146 (2014): 51-62.
- Liang Y, Mafuvadze B, Aebi JD, et al. Cholesterol biosynthesis inhibitor RO 48-8071 suppresses growth of hormone-dependent and castration-resistant prostate cancer cells. OncoTargets and Therapy 9 (2016): 3223- 3232.
- Mal R, Magner A, David J, et al. Estrogen receptor beta (ERβ): a ligand activated tumor suppressor. Front Oncol 10 (2020): 587386.
- Warner M, Gustafsson JA. The role of estrogen receptor beta (ERbeta) in malignant diseases—a new potential target for antiproliferative drugs in prevention and treatment of cancer. Biochem Biophys Res Commun 396 (2010): 63-66.
- Deroo BJ, Buensuceso AV. Minireview: estrogen receptorbeta: mechanistic insights from recent studies. Mol Endocrinol 24 (2010): 1703-171419-21.
- Mersereau JE, Levy N, Staub RE, et al. Cohen I, Bjeldanes LF, Leitman DC. Liquiritigenin is a plant-derived highly selective estrogen receptor beta agonist. Mol Cell Endocrinol 283 (2008): 49-57.
- Liu Y, Xie S, Wang Y, et al. Liquiritigenin inhibits tumor growth and vascularization in a mouse model of HeLa cells. Molecules 17 (2012): 7206-7216.
- Hodges-Gallagher L, Valentine CD, El Bader S, et al. Estrogen receptor beta increases the efficacy of antiestrogens by effects on apoptosis and cell cycling in breast cancer cells. Breast Cancer Res Treat. 109 (2008): 241-250.
- Alexandrova E, Lamberti J, Saggese P, et al. Small Non-Coding RNA Profiling Identifies miR-181a-5p as a Mediator of Estrogen Receptor Beta-Induced Inhibition of Cholesterol Biosynthesis in Triple-Negative Breast Cancer. Cells 9 (2020): 874.
- Alexandrova E, Giurato G, Saggese P, et al. Interaction Proteomics Identifies ERbeta Association with Chromatin Repressive Complexes to Inhibit Cholesterol Biosynthesis and Exert An Oncosuppressive Role in Triple-negative Breast Cancer. Mol Cell Proteomics 19 (2020): 245-260.
- Patch AM, Christie EL, Etemadmoghadam D, et al. Whole-genome characterization of chemoresistant ovarian cancer. Nature 521 (2015): 489-494.