Influence of the Fermentative Flora on the Carbohydrate content of Sorghum Grains
Article Information
Goubgou Mahamadé1,2*, Bationo Fabrice2, Nébié Sibiri1,2, Cissé Hama1, Songré-Ouattara T Laurencia2, Traoré Yves1, Savadogo Aly1
1University Joseph KI-ZERBO, Doctoral School of Sciences and Technology, Laboratory of Applied Biochemistry and Immunology (LABIA), 03 BP 7021 Ouagadougou 03, Burkina Faso
2Research Institute of Applied Sciences and Technology (IRSAT), Department of Food Technology (DTA), 03 BP 7047 Ouagadougou 03, Burkina Faso
*Corresponding Author: Goubgou Mahamadé, University Joseph KI-ZERBO, Doctoral School of Sciences and Technology, Laboratory of Applied Biochemistry and Immunology (LABIA), 03 BP 7021 Ouagadougou 03, Burkina Faso
Received: 01 February 2022; Accepted: 09 March 2022; Published: 11 Macrh 2022
Citation: Goubgou Mahamadé, Bationo Fabrice, Nébié Sibiri, Cissé Hama, Songré-Ouattara T Laurencia, Traoré Yves, Savadogo Aly. Influence of the Fermentative Flora on the Carbohydrate content of Sorghum Grains. Journal of Food Science and Nutrition Research 5 (2022): 351-357.
View / Download Pdf Share at FacebookAbstract
Microbial fermentation activates many endogenous enzymes that help improve the nutritional value of cereals. The present study aimed to determine the effect of the fermentation flora on the carbohydrate content of hulled and non-hulled sorghum grains fermented for 72 hours. The fermentation process, the enumeration of the total mesophilic aerobic flora, yeasts and molds and the total carbohydrate contents were made according to standard analysis methods. The pH of the fermentation waters was recorded every 24 hours. The results showed a significant drop in pH over fermentation time from 6.05 to 4.14 for hulled sorghum grains fermented for 72 hours. The evolution of loads in total mesophilic aerobic flora and yeasts and molds resulting in the drop in pH in unhulled sorghum grains is linked to alcoholic fermentation and that in hulled grains to lactic and acetic fermentation. The production of metabolites responsible for the drop in pH during fermentation would be linked to the degradation of carbohydrate compounds. Spontaneous fermentation of sorghum grains allowed the degradation of carbohydrate molecules and this could also be accompanied by an improvement in the bioavailability of nutrients contained in the grains.
Keywords
Sorghum grains, pH, Carbohydrates, Yeasts, Fermentation
Sorghum grains articles; pH articles; Carbohydrates articles; Yeasts articles; Fermentation articles
Sorghum grains articles Sorghum grains Research articles Sorghum grains review articles Sorghum grains PubMed articles Sorghum grains PubMed Central articles Sorghum grains 2023 articles Sorghum grains 2024 articles Sorghum grains Scopus articles Sorghum grains impact factor journals Sorghum grains Scopus journals Sorghum grains PubMed journals Sorghum grains medical journals Sorghum grains free journals Sorghum grains best journals Sorghum grains top journals Sorghum grains free medical journals Sorghum grains famous journals Sorghum grains Google Scholar indexed journals pH articles pH Research articles pH review articles pH PubMed articles pH PubMed Central articles pH 2023 articles pH 2024 articles pH Scopus articles pH impact factor journals pH Scopus journals pH PubMed journals pH medical journals pH free journals pH best journals pH top journals pH free medical journals pH famous journals pH Google Scholar indexed journals Carbohydrates articles Carbohydrates Research articles Carbohydrates review articles Carbohydrates PubMed articles Carbohydrates PubMed Central articles Carbohydrates 2023 articles Carbohydrates 2024 articles Carbohydrates Scopus articles Carbohydrates impact factor journals Carbohydrates Scopus journals Carbohydrates PubMed journals Carbohydrates medical journals Carbohydrates free journals Carbohydrates best journals Carbohydrates top journals Carbohydrates free medical journals Carbohydrates famous journals Carbohydrates Google Scholar indexed journals Yeasts articles Yeasts Research articles Yeasts review articles Yeasts PubMed articles Yeasts PubMed Central articles Yeasts 2023 articles Yeasts 2024 articles Yeasts Scopus articles Yeasts impact factor journals Yeasts Scopus journals Yeasts PubMed journals Yeasts medical journals Yeasts free journals Yeasts best journals Yeasts top journals Yeasts free medical journals Yeasts famous journals Yeasts Google Scholar indexed journals Fermentation articles Fermentation Research articles Fermentation review articles Fermentation PubMed articles Fermentation PubMed Central articles Fermentation 2023 articles Fermentation 2024 articles Fermentation Scopus articles Fermentation impact factor journals Fermentation Scopus journals Fermentation PubMed journals Fermentation medical journals Fermentation free journals Fermentation best journals Fermentation top journals Fermentation free medical journals Fermentation famous journals Fermentation Google Scholar indexed journals nutrients articles nutrients Research articles nutrients review articles nutrients PubMed articles nutrients PubMed Central articles nutrients 2023 articles nutrients 2024 articles nutrients Scopus articles nutrients impact factor journals nutrients Scopus journals nutrients PubMed journals nutrients medical journals nutrients free journals nutrients best journals nutrients top journals nutrients free medical journals nutrients famous journals nutrients Google Scholar indexed journals grains articles grains Research articles grains review articles grains PubMed articles grains PubMed Central articles grains 2023 articles grains 2024 articles grains Scopus articles grains impact factor journals grains Scopus journals grains PubMed journals grains medical journals grains free journals grains best journals grains top journals grains free medical journals grains famous journals grains Google Scholar indexed journals anti-nutritional factors articles anti-nutritional factors Research articles anti-nutritional factors review articles anti-nutritional factors PubMed articles anti-nutritional factors PubMed Central articles anti-nutritional factors 2023 articles anti-nutritional factors 2024 articles anti-nutritional factors Scopus articles anti-nutritional factors impact factor journals anti-nutritional factors Scopus journals anti-nutritional factors PubMed journals anti-nutritional factors medical journals anti-nutritional factors free journals anti-nutritional factors best journals anti-nutritional factors top journals anti-nutritional factors free medical journals anti-nutritional factors famous journals anti-nutritional factors Google Scholar indexed journals nutritional quality articles nutritional quality Research articles nutritional quality review articles nutritional quality PubMed articles nutritional quality PubMed Central articles nutritional quality 2023 articles nutritional quality 2024 articles nutritional quality Scopus articles nutritional quality impact factor journals nutritional quality Scopus journals nutritional quality PubMed journals nutritional quality medical journals nutritional quality free journals nutritional quality best journals nutritional quality top journals nutritional quality free medical journals nutritional quality famous journals nutritional quality Google Scholar indexed journals
Article Details
1. Introduction
Cereal raw materials contain anti-nutritional factors such as phytates, tannins, oxalates, alpha-galactosides and fiber [1,2]. In cereals, phytates normally form complexes with metal cations, including iron, zinc, calcium, magnesium and proteins in cereals. These complexes are usually degraded by enzymes, which require optimum pH [3]. Phytate is one of the greatest anti-nutrients, which is present in food crops [2]. Previous work has reported that high levels of anti-nutritional factors reduce mineral availability or absorption and could lead to nutrient deficiency or malnutrition [2,4]. Several treatments are used to eliminate or reduce anti-nutritional factors in foods. These include, among others, fermentation, steeping, malting, milling, roasting, pre-cooking, shelling, etc. [5,6]. Fermentation is an important process that significantly reduces the content of anti-nutritional factors such as phytic acid, tannins and polyphenols in cereals [7]. It is a metabolic process in which sugars are oxidized to produce energy. It activates many endogenous enzymes like phytase and also provides optimal pH conditions for the enzymatic degradation of phytate. Such a reduction in phytate can increase the amount of soluble iron, zinc, and calcium several-fold [8]. Tannin levels can be reduced through lactic fermentation and resulting in increased iron absorption [9]. Various previous studies have confirmed that fermentation is one of the best methods to reduce antinutritional factors in foods compared to all other methods and in turn improves the nutritional quality of foods. This concerns the increase in the content of essential amino acids such as lysine, methionine and tryptophan in the case of cereals [10]. It also improves the absorption of minerals from plant-based foods. During the fermentation process, several nutrients are needed and play an important role in the development of microorganisms. Among these nutrients, carbohydrates play an important role. The objective of this study was to follow the evolution of total mesophilic aerobic flora, yeasts and molds and total carbohydrate levels in hulled and non-hulled sorghum grains fermented for 24, 48 and 72 hours.
2. Material and methods
2.1 Fermentation of sorghum grains
The fermentation of the grains was carried out according to a process adapted to that described by Adebiyia et al. [11]. Hulled and unhulled white sorghum grains were washed and soaked in distilled water (1:4 w/v) at room temperature for 24, 48 and 72 hours. The grains were then washed with tap water, rinsed with distilled water and dried in a hot air oven at 40°C for 24 hours. The dried grains were ground dry in a mill and sieved using a sieve with a mesh size of less than 200 mm to obtain a fine fermented flour. The flours were packed in plastic bags and kept at room temperature before the analyses. Each fermentation was carried out in triplicate.
2.2 Evaluation of the microbial load
Microbiological analyses of fermented juice were performed according to standard methods described in the manual of microbiological analysis. The bacterial populations in the fermented juice were enumerated after prepared stock solution and decimal dilutions according to standard microbial methods. 10 mL of the sample were added to 90 mL of sterile buffered peptone water, and serial dilutions were monitored with this suspension. All tests were done in duplicate. The results were expressed as colony-forming unit per milliliter (CFU/mL). Total microbial flora was enumerated on plate count agar after incubation at 30°C during 24-48 h. Yeasts and molds were enumerated on Sabouraud CAF agar with chloramphenicol, after incubation at 25°C for 3-5 days.
2.3 Determination of sample pH
The pH was determined according to the potentiometric method (AOAC 943.02 1990). A volume of 50 mL of distilled water was added to 10 g of sample contained in a 250 mL Erlenmeyer flask. After stirring, the pH was measured on the homogeneous mixture obtained using a pH meter, brand WTW pH 340.
2.4 Dosage of total sugars
The total sugars were determined by the orcinol method described by Montreuil and Spik [12]. Indeed, under the action of concentrated sulfuric acid (95-98%) and at hot, the hexoses and pentoses in the medium undergo extensive internal dehydration, followed by cyclization leading to the formation of derivatives of furfural and 5-hydroxymethylfurfural, reacting with orcinol. The formation of a yellow-red complex makes it possible to monitor the concentration of total sugars in the sample by reading the absorbance at 510 nm. To do this, 0.05 g of crushed sample was taken into a beaker and 5 ml of distilled water was added to it. The mixture obtained was subjected to magnetic stirring for 10 min, then transferred to a 100 ml volumetric flask and the volume completed with distilled water. After homogenization, 1 ml of the mixture was introduced into a test tube, and added with 2 ml of orcinol reagent and 7 ml of 60% H2SO4 and this in duplicate. The test tubes were shaken and then brought to a boiling water bath for 20 min. They were then placed in the dark for 45 min and then at room temperature for 10 min. After homogenization, the absorbance was measured at 510 nm on a spectrophotometer. The total sugars content was determined from the D-glucose calibration curve already established.
3. Results and discussion
3.1 Evolution of pH during fermentation
The results of the evolution of the fermentation pHs are presented in figure 1. The pHs of the fermentation media all evolved in a decreasing manner during the fermentation time. Thus, the lowest pH was obtained with the fermentation of shelled grains after seventy-two (72) hours, i.e. a pH of 4.14. The highest pH was 6.06 and was obtained with shelled grains at T0. The statistical analysis showed that the initial pHs were not significantly different from each other but a significant difference was observed after 24 hours of fermentation, reflected by a lowering of the pH. The drop in pH reflects an acidification of the fermentation media and could be explained either by microbial activity or by enzymatic degradation. These results on the evolution of the pH are in agreement with those of Bokossa Yaou et al. [13] and Banon et al. [14] on other fermented products. These authors explained that the drop in pH during fermentation was characteristic of cereals and would be linked to an increase in the number of lactic acid bacteria [13,14]. The work of Banou et al. showed a drop in pH from 5.40 ± 0.11 to 3.27 ± 0.12 during fermentation of millet and sorghum.
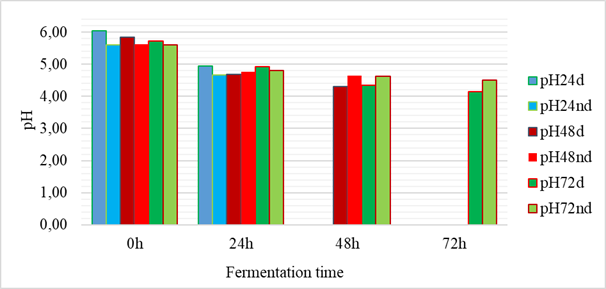
Figure 1: Evolution of pH during fermentation (24h, 48h, 72h: fermentation time; d: hulled grains; nd: unshelled grains)
3.2 Evolution of FAMT and yeasts and molds during fermentation
After each fermentation, the water used was collected to count the total mesophilic aerobic flora and the yeasts and molds. The results of the FAMT count are presented in figure 2. For the non-husked samples, an increase in the FMAT was observed with the fermentation time, i.e., a change of 4.20.1008 CFU/mL (after 24 hours of fermentation) at 2.86.109 CFU/ml (after 72 hours of fermentation). This increase in FAMT is similar to the results reported by Banon et al. from the fermentation of millet and sorghum. On the other hand, at the level of the fermentation solutions of the shelled grains, we note a decrease in the load of FAMT. This could be explained by the acidification of the environment, therefore the influence of the drop in pH. The fermentation medium of the non-hulled grains was therefore favorable to the development of FAMT and that of the hulled grains was unfavorable to it due to an intense activity of the fermentation flora. Hulled kernels had a high FAMT load at steeping compared to unhulled kernels. This suggests a strong contamination by FAMT during the shelling process.

Figure 2: Evolution of FAMT during fermentation
3.3 Evolution of yeasts and molds during fermentation
The results of the counting of yeasts and molds are presented in figure 3. For the non-husked samples, a decrease with the fermentation time was observed. Thus, the fungal flora load went from 3.8×104 CFU/mL to 1.85×104 CFU/mL. On the other hand, at the level of the fermentation solutions of the hulled grains, an increase in yeasts and molds was observed. The load went from 1.24.104 CFU/mL to 1.04.105 CFU/mL. The fermentation medium of unshelled grains was therefore unfavorable to the growth of yeasts and molds, but unlike that of shelled grains. Unshelled grains also presented a higher number of yeasts and molds on soaking compared to shelled grains.

Figure 3: Evolution of yeasts and molds during fermentation
3.4 Variation in the carbohydrate content in the grains
The variation in the carbohydrate content in the grains is recorded in Table 1. The lowest carbohydrate levels having been obtained with unhusked sorghum grains fermented for 72 hours, i.e., 73.7% relative to the dry matter. In both types of grains (shelled and unshelled), a decrease in total sugars was observed compared to unfermented control grains (ND-NT and D-NT). This variation is significant (p < 0.05) for shelled grains. This reduction in carbohydrate content is due to the use of carbohydrates by microorganisms for their metabolic functions. This results in the increase of the microbial population and the production of secondary metabolites such as lactic acid, ethanol, acetic acid, bacteriocins, reuterin, reutericycline, hydrogen peroxides, fatty acids in the fermentation medium. These metabolites are responsible for inhibiting pathogens and preserving food. Note that the fermentation metabolism of yeast mainly leads to the production of CO2 and ethanol but to other compounds such as carboxylic acids, esters and aldehydes giving cereal seeds and their derivatives interesting organoleptic characteristics.
Total sugar |
|
ND-NT |
75.19 a, b, c |
D-NT |
82.43 e |
ND -F24 |
73.28 a |
ND -F48 |
74.07 a, b |
ND -F72 |
73.7 a, b |
D-F24 |
78.48 d |
D-F48 |
77.58 c, d |
D-F72 |
80.15 d, e |
Table 1 : Total sugar content of the different samples
4. Conclusion
This study has shown the importance of bacterial and fungal flora during the fermentation of cereal grains. This fermentation is initiated by bacteria and continues by yeasts and moulds. Thus, giving the flour obtained after grinding the seeds special characteristics.
References
- General standard for food additives CODEX STAND 192-1995. In Codex Alimentarius International Food Standards (2019).
- Samtiya M, Aluko RE, Dhewa T. Plant food anti-nutritional factors and their reduction strategies?: An overview 5 (2020): 1-14.
- Gibson RS, Bailey KB, Gibbs M, et al. A review of phytate, iron, zinc, and calcium concentrations in plant-based complementary foods used in low-income countries and implications for bioavailability. Food and Nutrition Bulletin 31 (2010): 134-146.
- Galati A, Oguntoyinbo FA, Moschetti G, et al. The cereal market and the role of fermentation in cereal-based food production in Africa. Food Reviews International 30 (2014): 317-337.
- Foisnet E. Acide phytique: fermentation et réduction de la quantité d’acide phytique dans les produits alimentaires à base de céréales ou de protéagineux (2010).
- Handa V, Kumar V, Panghal A, et al. Effect of soaking and germination on physicochemical and functional attributes of horsegram flour. Journal of Food Science and Technology 54 (2017) 4229-4239.
- Simwaka JE, Chamba MVM, Huiming Z, et al. Effect of fermentation on physicochemical and antinutritional factors of complementary foods from millet, sorghum, pumpkin and amaranth seed flours. In International Food Research Journal 24 (2017): 1869-1879.
- Gupta RK, Gangoliya SS, Singh NK. Reduction of phytic acid and enhancement of bioavailable micronutrients in food grains. Journal of Food Science and Technology 52 (2013): 676-684.
- Ray RC, Montet D. Microorganisms and fermentation of traditional foods (C. Press & T. & F. Group (eds.), (2015).
- Mohapatra D, Patel AS, Kar A, et al. Effect of different processing conditions on proximate composition, anti-oxidants, anti-nutrients and amino acid profile of grain sorghum. Food Chemistry 271 (2019): 129-135.
- Adebiyi JA, Obadina AO, Adebo OA, ET AL. Comparison of nutritional quality and sensory acceptability of biscuits obtained from native, fermented, and malted pearl millet (Pennisetum glaucum) flour. Food Chemistry 232 (2017): 210-217.
- Montreuil J, Spik G. Micro dosage des glucides, méthodes colorimétriques de dosage des glucides totaux (1969).
- Bokossa Yaou I, Banon JSB, Tchekessi CCK, et al. Production de Ablo , un aliment céréalier fermenté du Bénin: Synthèse bibliographique. International Journal of Innovation and Applied Studies 16 (2016): 356-369.
- Banon SBJ, Bokossa YIP, Tchekessi CKC, et al. Microbiological and physico-chemical dynamics during the fermentation of the millet-based (Pennisetum glaucum) Ablo and the sorghum-based (Sorghum bicolor) Ablo in the Republic of Benin. International Journal of Biomolecules and Biomedicine 6 (2017): 1-9.