Essential Metal-Based Nanoparticles (Copper/Iron NPs) as Potent Nematicidal Agents against Meloidogyne spp.
Article Information
Gkanatsiou Ch1, Ntalli N2, Menkissoglu-Spiroudi U3, Dendrinou-Samara C1*
1Inorganic Chemistry Lab, Chemistry Department, Aristotle University of Thessaloniki, Thessaloniki, Greece
2Benaki Phytopathological Institute, Department of Pesticides’ Control and Phytopharmacy, Athens, Greece
3Pesticide Science Laboratory, School of Agriculture, Faculty of Agriculture, Forestry and Environment, Aristotle University of Thessaloniki, Thessaloniki, Greece
*Corresponding Author: Dendrinou-Samara C, Inorganic Chemistry Lab, Chemistry Department, Aristotle University of Thessaloniki, Greece, 54124 Thessaloniki, Greece
Received: 01 July 2019; Accepted: 15 July 2019; Published: 24 July 2019
Citation:
Gkanatsiou Ch, Ntalli N, Menkissoglu-Spiroudi U, Dendrinou-Samara C. Essential Metal-Based Nanoparticles (Copper/Iron NPs) as Potent Nematicidal Agents against Meloidogyne spp. Journal of Nanotechnology Research 1 (2019): 044-058.
View / Download Pdf Share at FacebookAbstract
Root-knot nematodes (Meloidogyne spp.) cause great economic impact in the sector of agriculture while the number of existing commercial nematicides has been drastically reduced. The metal-based nanoparticles offer new possibilities as agrochemicals. In the current study, we shed light on the role of copper/iron based nanoparticles as bioactive agents against the root knot nematode Meloidogyne spp; along with the plant enhancement properties on the nematode infested host. Same sized PEGylated Cu, CuFe and CuFeO2 NPs, were solvothermally synthesized characterized and evaluated against M. incognita and M. javanica nematodes, in terms of paralysis and biological cycle arrest in tomato plants. Comparisons were made against the commercial nematicide fosthiazate. All the tested compositions revealed nematicidal activity, but CuFe NPs were found best effective exhibiting the lowest EC50 value calculated at 0.03 μg ai g/soil. Additionally, a fresh shoot and root weight increase was evident in tomato plants treated with CuFe NPs.
Keywords
Meloidogyne incognita, Meloidogyne javanica, Micronutritients, Nanoparticles, Nanoagrochemicals, Plant protection, Fertilizers
Meloidogyne incognita articles Meloidogyne incognita Research articles Meloidogyne incognita review articles Meloidogyne incognita PubMed articles Meloidogyne incognita PubMed Central articles Meloidogyne incognita 2023 articles Meloidogyne incognita 2024 articles Meloidogyne incognita Scopus articles Meloidogyne incognita impact factor journals Meloidogyne incognita Scopus journals Meloidogyne incognita PubMed journals Meloidogyne incognita medical journals Meloidogyne incognita free journals Meloidogyne incognita best journals Meloidogyne incognita top journals Meloidogyne incognita free medical journals Meloidogyne incognita famous journals Meloidogyne incognita Google Scholar indexed journals Meloidogyne javanica articles Meloidogyne javanica Research articles Meloidogyne javanica review articles Meloidogyne javanica PubMed articles Meloidogyne javanica PubMed Central articles Meloidogyne javanica 2023 articles Meloidogyne javanica 2024 articles Meloidogyne javanica Scopus articles Meloidogyne javanica impact factor journals Meloidogyne javanica Scopus journals Meloidogyne javanica PubMed journals Meloidogyne javanica medical journals Meloidogyne javanica free journals Meloidogyne javanica best journals Meloidogyne javanica top journals Meloidogyne javanica free medical journals Meloidogyne javanica famous journals Meloidogyne javanica Google Scholar indexed journals Micronutritients articles Micronutritients Research articles Micronutritients review articles Micronutritients PubMed articles Micronutritients PubMed Central articles Micronutritients 2023 articles Micronutritients 2024 articles Micronutritients Scopus articles Micronutritients impact factor journals Micronutritients Scopus journals Micronutritients PubMed journals Micronutritients medical journals Micronutritients free journals Micronutritients best journals Micronutritients top journals Micronutritients free medical journals Micronutritients famous journals Micronutritients Google Scholar indexed journals Nanoparticles articles Nanoparticles Research articles Nanoparticles review articles Nanoparticles PubMed articles Nanoparticles PubMed Central articles Nanoparticles 2023 articles Nanoparticles 2024 articles Nanoparticles Scopus articles Nanoparticles impact factor journals Nanoparticles Scopus journals Nanoparticles PubMed journals Nanoparticles medical journals Nanoparticles free journals Nanoparticles best journals Nanoparticles top journals Nanoparticles free medical journals Nanoparticles famous journals Nanoparticles Google Scholar indexed journals Nanoagrochemicals articles Nanoagrochemicals Research articles Nanoagrochemicals review articles Nanoagrochemicals PubMed articles Nanoagrochemicals PubMed Central articles Nanoagrochemicals 2023 articles Nanoagrochemicals 2024 articles Nanoagrochemicals Scopus articles Nanoagrochemicals impact factor journals Nanoagrochemicals Scopus journals Nanoagrochemicals PubMed journals Nanoagrochemicals medical journals Nanoagrochemicals free journals Nanoagrochemicals best journals Nanoagrochemicals top journals Nanoagrochemicals free medical journals Nanoagrochemicals famous journals Nanoagrochemicals Google Scholar indexed journals Plant protection articles Plant protection Research articles Plant protection review articles Plant protection PubMed articles Plant protection PubMed Central articles Plant protection 2023 articles Plant protection 2024 articles Plant protection Scopus articles Plant protection impact factor journals Plant protection Scopus journals Plant protection PubMed journals Plant protection medical journals Plant protection free journals Plant protection best journals Plant protection top journals Plant protection free medical journals Plant protection famous journals Plant protection Google Scholar indexed journals Fertilizers articles Fertilizers Research articles Fertilizers review articles Fertilizers PubMed articles Fertilizers PubMed Central articles Fertilizers 2023 articles Fertilizers 2024 articles Fertilizers Scopus articles Fertilizers impact factor journals Fertilizers Scopus journals Fertilizers PubMed journals Fertilizers medical journals Fertilizers free journals Fertilizers best journals Fertilizers top journals Fertilizers free medical journals Fertilizers famous journals Fertilizers Google Scholar indexed journals synthetic carbamate articles synthetic carbamate Research articles synthetic carbamate review articles synthetic carbamate PubMed articles synthetic carbamate PubMed Central articles synthetic carbamate 2023 articles synthetic carbamate 2024 articles synthetic carbamate Scopus articles synthetic carbamate impact factor journals synthetic carbamate Scopus journals synthetic carbamate PubMed journals synthetic carbamate medical journals synthetic carbamate free journals synthetic carbamate best journals synthetic carbamate top journals synthetic carbamate free medical journals synthetic carbamate famous journals synthetic carbamate Google Scholar indexed journals organophthalide articles organophthalide Research articles organophthalide review articles organophthalide PubMed articles organophthalide PubMed Central articles organophthalide 2023 articles organophthalide 2024 articles organophthalide Scopus articles organophthalide impact factor journals organophthalide Scopus journals organophthalide PubMed journals organophthalide medical journals organophthalide free journals organophthalide best journals organophthalide top journals organophthalide free medical journals organophthalide famous journals organophthalide Google Scholar indexed journals environment articles environment Research articles environment review articles environment PubMed articles environment PubMed Central articles environment 2023 articles environment 2024 articles environment Scopus articles environment impact factor journals environment Scopus journals environment PubMed journals environment medical journals environment free journals environment best journals environment top journals environment free medical journals environment famous journals environment Google Scholar indexed journals
Article Details
1. Introduction
Root-knot nematodes (Meloidogyne spp.) are the most notorious plant parasitic nematodes, amongst agricultural pests suppressing crops while causing annually significant crop losses to the production of fruits and vegetables [1, 2]. Till now, nematicidal control has been mainly based on synthetic organic chemical nematicides [3]. However, due to environmental problems, many of them such as the synthetic carbamate, organophosphate and organophthalide pesticides have been recently banned (Council Directive 91/414/EEC, Regulation 2009/1107/EC OL and Directive 2009/128/EC). Therefore, there is an emergent need for the development of alternative nematode control measures and green nematicidal products that will impose less toxicity on non-target organisms, environment and humans [4].
Nanoagriculture involves the employment of nanomaterials, such as nanopesticides and nanofertilizers, in crop protection, providing sustainable options to improve plant’s health [5-8]. In recent years, among a variety of nanostructures, metal-based nanoparticles (NPs) are gaining interest due to their unique physicochemical properties and biological activities. The impact of metal-based NPs on plant growth and their accumulation in food source according to the applied dose are evident. The effectiveness of metal-based NPs are species dependent and is driven by a series of factors, including the characteristics of the nanomaterial and the environmental conditions. The physicochemical properties of the NPs, known as the so-called 4s' parameters (size, shape, surface chemistry and structure), can highly affect their biological properties; while the environmental behaviour of the NPs (mobility, reactivity, toxicity) needs to be completely understood. Thus, the effects of metal-based NPs formulations in different soils and on diverse crop plants have to be explored to broaden the horizon of sustainable agricultural production of higher and safer yields [6].
Copper (Cu) and iron (Fe) are essential micronutrients in crop plants and play a very important role in biochemical functions such as the photosynthetic electron transport, the respiration of the mitochondria, the oxidative stress, the metabolism of the cell wall and the synthesis of the DNA [5, 6]. At the same time, copper formulations can be used as antagonists of plant antagonistic bacteria and fungi, with huge potential for use in pesticide formulations [2, 4]. Interestingly, Fe-based NPs exhibit great potential in the agricultural section as they are considered to be biocompatible and of low-toxicity [9]. Additionally, iron deficiency is a widespread agronomic issue caused by poor iron solubility in the vast majority of soils and consequential insufficient iron availability to plants [10]. Fe-based NPs are reported to have insignificant antimicrobial activity against many bacteria species, such as Bacillus subtilis and Escherichia coli [11] and their use is primarily as fertilizers. In fact, naked iron oxide (Fe3O4) NPs were found to increase the shoot and root length of tomato plants [12] while the application of Fe2O3 NPs to peanut seeds, resulted in stem and root growth and seed germination increase, indicating that Fe2O3 NPs can replace traditional Fe fertilizers in the cultivation [13].
On the other hand, Cu-based NPs reveal their effectiveness and specificity towards a broad spectrum of microorganisms [14, 15]. It is a matter of concern, though, which form of copper (Cu, Cu2O, CuO) is more suitable, since it is linked to the flexible Cu(I)/Cu(II) redox activity and may undergo chemical transformations such as reduction or oxidation when applied to the soil [16]. However, the application of nanomaterials hardly reaches field experiments [17, 18] and the impact/ interaction of metal and metal oxide NPs on plants and soil are often controversial. The toxicity of the NPs in plants and the environment is correlated to the size, concentration and composition. In general, copper oxide NPs (CuO) are reported to be more toxic on plants, than metallic copper NPs, due to oxidative properties [19]. For instance, naked CuO NPs, of size <50 nm, prevented the growth of wheat in the sand when tested at concentrations >10 mg CuO/kg [20]; while in the case of naked Cu NPs of 20 nm a dose dependent decrease occurred in mung bean and wheat growth at concentrations >200 mg/L and >800 mg/L respectively [21]. On the other hand, CuO NP were found to have a positive impact on waterweed aquatic plants (Elodea densa), by stimulating photosynthesis even at low concentrations <0.25 mg/L [19]. Meanwhile, studies concerning the interaction of Cu2O NPs with soil and plants have not been reported. Specifically, applications of metal- based NPs, as nematicidal agents are very few. When ‘naked’ silver NPs were tested at 200 mg/mL against Meloidogyne incognita J2 100% immobility and mortality was revealed, while treatment at 0.0007% (w/w) of nano-silver completely controlled M. incognita in pot experiments; on the contrary, titanium oxide NPs tested at 800 and 400 mg/mL provoked 4.3 and 2% J2 mortality, respectively [22]. Interestingly, in other studies, when M. incognita J2 were immersed into 30-150 μg/mL of silver NPs in water, >99% nematodes became inactive in 6 hours [23].
Previously we have reported on the enhanced antimicrobial activity of CuFeO2 against Bacillus subtilis, Escherichia coli and Saccaromyces cerevisiae [24]. The composition of CuFeO2 as a bimetallic oxide with an inert iron (III) and a reactive copper (I) metals in the presence of the biodegradable polyethylene glycol (PEG8000), helping avoid oxidation of the metal core and to protect plant cells from possible increased NPs toxicity by performing stealth properties [25], sounds promising. In continuing our studies in the field of nanoagrochemicals [16, 24, 26], we report on different species of copper/ iron-based NPs activity as nematicidals for the first time. In particular, in this study we report results on (1) the synthesis and characterization of the same crystallite sized Pegylated Cu, CuFe and CuFeO2 NPs prepared solvothermally in the presence of the biodegradable polyethylene glycol (PEG8000) and (2) evaluation of the nematicidal activity of all tested compositions against M. incognita and M. javanica both in terms of J2s paralysis and biological cycle arrest of the parasite in host plant tissues. Last we report on the secondary effects on tomato plants’ growth, by counting fresh shoot and root weight.
2. Materials and Methods
2.1 Reagents
The reagents that were necessary for the preparation of nanoparticles are the following: Copper(II) nitrate trihydrate Cu(NO3)2·3H2O (Merck, ≥ 99.5%, M=241.60 g/mol), Iron(III) nitrate nonahydrate Fe(NO3)3·9H2O (Merck, ≥ 99.5%, M=404 g/mol), polyethylene glycol (PEG) 8000 (Alfa aesar), hydrazine hydrate N2H4·H2O (Merck, about 100%, M=50.06 g/mol).
2.2 Preparation of Cu-based NPs
Cu NPs: 1 g (4 mmol) of Cu(NO3)2·3H2O was dissolved in 6 mL of deionized water to obtain a blue solution. Then 0.1g (20.5 mmol) of N2H4·H2O was added dropwise to the blue solution and the color changed to yellow, indicating the reduction of Cu (II). Successively, 1.5 g (1.9 mmol) of PEG 8000 was added. The resulting solution was stirred thoroughly and then transferred into a 23 mL Teflon-lined stainless-steel autoclave. The crystallization was carried out for 2 h, under autogenous pressure, at the temperature of 160°C. Then the autoclave was cooled naturally to room temperature. The supernatant liquid was discarded after centrifugation at 5000 rpm. A black-brown precipitate was obtained and washed at least three times with ethanol, to remove the excess of ligands and the unreacted precursors.
CuFe and CuFeO2 NPs: For the preparation of these compositions a modified synthetic protocol of our previous studies was followed according [27]. In brief for CuFe NPs preparation: Fe(NO3)3·9H2O (0.5 mmol, 0.2 g) and Cu(NO3)2·3H2O (0.5 mmol, 0.1 g) were dissolved in 8 mL of TEG, while for CuFeO2 NPs: 0.5 mmol (0.2 g) Fe(NO3)3·9H2O and 0.1 g (0.5 mmol) of Cu(NO3)2·3H2O were dissolved in 8 mL deionized water. Then 0.1 g (39.5 mmol) of N2H4·H2O was added dropwise to this solution and the color changed from yellow to brown. Successively a solution of 1.9 mmol (1.5 g) PEG 8000 was added. In both cases the resulting solution was stirred thoroughly and then transferred into a 23 mL Teflon-lined stainless-steel autoclave. The crystallization was carried out for 8 h, under autogenous pressure, at the temperature of 160°C. Then the autoclave was cooled naturally to room temperature. The supernatant liquid was discarded after centrifugation at 5000 rpm. A black-brown precipitate was obtained and washed at least three times with ethanol, to remove the excess of ligands and the unreacted precursors.
Composition |
Surfactant (%) |
Size by XRD (nm) |
Size by DLS (nm) |
Cu |
40% PEG8000 |
40 |
200 |
CuFe |
39% PEG8000 |
40 |
146 |
CuFeO2 |
20% PEG8000 |
36 |
257 |
Table 1: Experimental results and main characteristics of Cu, CuFe and CuFeO2 NPs.
2.3 Ionic release measurements
The release of copper and iron ions from the Cu, CuFe and CuFeO2 NPs into distilled (DI) water was studied by preparing a solution of 10 mg of NPs in 100 mL DI water. The solution was sonicated for 10 min. The suspension was kept in a rotary shaker under the same experimental conditions as in paralysis bioassays for 24 h and then the residual Cu2+ and Fe2+ concentration in the aqueous phase was determined using Inductively Coupled Plasma Atomic Emission Spectroscopy (ICP-AES).
2.4 Dynamic light scattering measurements
Hydrodynamic diameters were determined with VASCO Flex™ Particle Size Analyzer NanoQ V2.5.4.0 dynamic light scattering (DLS), by electrophoretic measurements, at 25°C. For the measurements of all the samples, the dilution medium was filtered through a 0.2-micron membrane, at the concentration of 100 μg/mL because a high purity of the suspension was required in order to remove unwanted agglomerates and/or dust contamination.
2.5 NPs characterization
The characterization of the NPs was performed by X-ray powder diffraction (XRD) on a Philips PW 1820 diffractometer at a scanning rate of 0.050/3 s, in the 2θ range from 10 to 90°, with monochromatized Cu Kα radiation (λ=1.5406 nm). TG analysis (SETA-RAM SetSys-1200) was carried out in the range from room temperature to 900°C at a heating rate of 10°C min− 1 under N2 atmosphere, by Infrared spectra (280-4000 cm−1) were recorded on a Nicolet and with FTIR 6700 spectrometer with samples prepared as KBr pellets.
2.6 Nematode bioassays
2.6.1 Nematode cultures: Two separate nematode species were raised by a single egg mass of M. incognita and another of M. javanica as characterized and kindly provided from the collection of E. A. Tzortzakakis of Hellenic Agricultural Organization-Demeter, Heraklion, Crete (ELGO). The obtained populations were reared on six-leaf stage tomato plants cv. Belladonna. Forty days after inoculation the roots were washed free of soil, cut into 2 cm pieces and eggs were extracted from a tomato plant roots, according to the sodium hypochlorite procedure [28] as described by Ntalli et al. [29]. Second-stage juveniles (J2) were allowed to hatch in modified Baermann funnels at 27oC. The first 3-day (J2) hatching was discarded. All freshly hatched (24 h) second-stage juveniles (J2) collected thereafter were used in the experiments.
2.6.2 Effect of NPs on J2 motility: Effects of Cu and CuFeO2 or CuFe NPs on J2 motility, were tested at the dose rate of 25-200 μg/mL or 12.5-200 μg/mL, respectively, in CellstarR 96-well cell culture plates (Greiner bio-one). In all cases, NPs were dispersed in water to form test solutions, which were mixed in 96-well culture plates at a ratio of 1:1 v/v with suspensions of 20 J2 per well. Distilled water was used as control. All the plates were covered with plastic lids and kept in the dark at 27°C. The exact number of transferred J2 nematodes into the wells, was verified under an inverted microscope (Euromex, The Netherlands) at 40X. After 24, 48 and 72 h the mobile and immobile Juveniles (J2) were observed with the aid of the inverted microscope. The five treatment replications were completely randomized and each experiment was performed twice.
2.6.3 The NPs effect on the nematode’s life cycle: The soil that was used for the pot experiments was an RKN-free clay loam soil containing 1.3% organic matter-pH 7.8 and was collected from a non-cultivated field of the University Farm. A 3-mm sieve was used in order to separate soil from debris. The sieved soil was then partially air-dried overnight. Measurements of soil moisture and maximum water holding capacity (MWHC) were performed according to Pantelelis et al. [30]. After the confirmation of the absence of nematodes using the modified Baermann funnel method, the soil was mixed with sand at a ratio of 2:1 to obtain the mixture hereafter referred to as soil. Plastic bags of 1.0-kg subsamples were prepared representing the experimental treatments. 12.500 J2 were applied to each plastic bag and the soil was mixed thoroughly by shaking, then sieved and equilibrated in the dark, for 24 h, at room temperature. The fortification of the soil with NPs took place at doses of 0.025 to 0.4 μg ai/gr soil. For this, appropriate amounts of CuFe, CuFeO2 and Cu NPs were weighed, dispersed in distilled water and the produced solutions (10 mL each) were mixed with the soil so as to achieve the test concentrations.
A treatment with water and a treatment with Nemathorin150EC at the registered dose, 2 μg ai/gr soil (a.i. fosthiazate 15%, Hellafarm Co.) were used as controls. The soil in the plastic bags was mixed and sieved again, through a 3-mm sieve, to ensure uniform distribution and then returned into the plastic bags, mixed thoroughly by shaking and left to equilibrate in the dark, for another 24 h, at room temperature. The moisture content of the soil never exceeded 24% of MWHC. After sieving again, the treated soil was divided into 200 g/ plastic pots (1kg × 5 treatments) and tomato plants cv. Belladonna were transplanted. Plants used for the experiment were 7-week old and at the six-leaf stage. The plants were watered every two days with 20 mL of water and were kept at 27°C, 60% RH at 16 h photoperiod. After forty days, the plants were uprooted and their roots were washed very gently in running tap water to remove all soil particles. In order to count nematode females in plant tissues, they were stained with an acid fuchsin solution [31]. The number of female nematodes per gram of root were counted at 10 × magnification under uniform illumination by transparent light within tissue sample. Measurements of fresh root and fresh shoot weight parameters were performed as well. The experiment was replicated once, and the treatments were always arranged in a completely randomized design with five replicates.
2.7 Statistical analysis
Nematode bioassays were performed twice with five replicates per treatment. Because ANOVA indicated no significant treatment by time interaction (between runs of experiment), means were averaged over the experiments and the data were analyzed (ANOVA) combined over time. The percentages of immotile J2 in the microwell assays were expressed as a percentage increase in the number of immotile J2 in the water control according to the Schneider Orelli’s formula: corrected % = {[mortality % in treatment − mortality % in control]/[100 − mortality % in control]} × 100 [32]. Data was subjected to probit linear regression analysis and the concentration of nanoparticles (EC50 values) required for 50% death/paralysis of nematodes was calculated. The data from the pot experiments were expressed as a percentage decrease in the number of females per gram of root corrected according to the control, using the Abbott’s formula: corrected% = 100 × {1−[females number in treated plot/females number in control plot]}. A probit linear regression analysis was performed for EC50 calculation. In this regression equation, the test compounds was the independent variable (x) and the number of female nematodes percentage decrease over water control, was the dependent variable (y). Treatments means for plant growth parameters were compared using Tukey’s test at P=0.05 [33]. Statistical analysis was performed using IBM SPSS Statistics program while the dose-response curves were created using the program Origin Pro 8 (Graphing and Analysis).
3. Results
3.1 Characterization of Cu-based NPs
In the XRD patterns of the samples studied (Figure 1) the Bragg reflections correspond to the crystal structures of Cu, CuFe and CuFeO2 NPs with Cards pdf.04-0836, pdf.03-065-7002 and pdf.75-2146 respectively. The reflections at almost 18,5° and 19,1° are associated with the presence of the organic coating (PEG8000) in Cu and CuFe NPs revealing the high crystallinity of the NPs. MDI's Jade software was used and lattice parameters were calculated. They were found to be a = 3.59 Å, 3.6170 Å, 3.05 for Cu and CuFe, respectively. For the rhombohedral structure of CuFeO2 the lattice parameters were found to be a = 3.04Å and c = 17.12Å. The average crystalline sizes of pure Cu, CuFe and CuFeO2 NPs were determined by using the Scherrer equation and taking the full width at half maximum (fwhm) of the most intense peak and were found to be 40 nm, 40 nm and 36 nm respectively.
FTIR spectra of Cu, CuFe and CuFeO2 NPs with the corresponding pattern of PEG8000 is given in Figure 2. The surfactant PEG8000 was confirmed by the comparison of the two IR patterns in each sample. Peaks at 2880 cm−1 are assigned to the stretching vibrations of methylene groups (-CH2), in the surfactant molecules. There is a broad peak around 1635 cm−1 ascribed to the hydrogen bonds which is formed from the hydroxide groups. The C-O-C ether stretch band at 1110 cm−1 is indicative of the presence of PEG8000 on the samples. The vibrations around 1340 cm-1 and 1470 cm-1 are associated with (C-H) and (C-H2) bending respectively. Finally, in the case of CuFeO2 NPs we can observe a sharp peak at 424 cm-1 indicating the metal oxide nature of the NPs. The amount of the surfactants on the samples was evaluated by thermogravimetric analysis (TGA). Under nitrogen atmosphere the temperature range was high as 800°C. The percentage of the cumulative organic content was estimated at 40, 39 and 20 wt% for the PEG8000- capped Cu, CuFe and CuFeO2 NPs respectively (Figure 3).
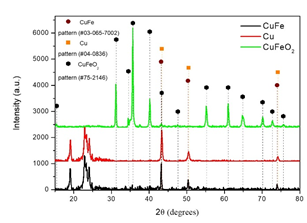
Figure 1: XRD patterns of Cu, CuFe, CuFeO2 NPs.
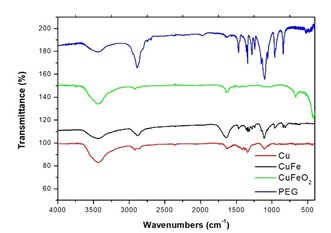
Figure 2: FTIR spectra of the samples Cu, CuFe, CuFeO2 NPs.

Figure 3: Thermogravimetric curves of Cu, CuFe and CuFeO2 NPs.
3.2 Ion release measurements
The stability of the NPs was investigated in aqueous suspensions and the ions released from Cu, CuFe and CuFeO2 NPs into the water were estimated after 24 h. The concentration of copper ions released in water after 24 h was found at 19.8, 13.3 and 1.6 μg/mL for CuFe, CuFeO2, Cu, respectively (Table 2). Οn the contrary, release of Fe ions was found low at 0.5 and 0.2 μg/mL for CuFe and CuFeO2 respectively.
Sample |
Cu |
Fe |
Cu |
1.6 |
- |
CuFe |
19.8 |
0.5 |
CuFeO2 |
13.3 |
0.2 |
Table 2: Concentrations (μg/mL) of Cu and Fe ions in water after 24 h dispersion of Cu-based NPs (100 μg/mL) in water.
3.3 Effect of NPs on J2 motility
The effect of Cu, CuFe, CuFeO2 NPs on the motility of root-knot nematodes was evaluated. When the NPs were tested against M. incognita and M. javanica, a clear dose response relationship was established and after 24 h a significant paralysis of J2 was evident (Figure 4). The dose response curves of Cu, CuFe, CuFeO2 NPs after 24 h of J2 immersion in NPs solutions are presented in Figure 4. The calculated EC50 values for all tested NPs against both nematode species after J2 immersion for 24, 48 and 72 h, are shown in Table 3. Among the three tested compositions, CuFe NPs exhibited the highest nematicidal activity, causing 100% paralysis to both Meloidogyne species, after 72 h of J2 exposure at the lowest dose. The EC50 values of Cu, CuFe and CuFeO2 NPs, after 24 h immersion of M. incognita J2 in test solutions, was calculated at 75, 18 and 73 μg/mL respectively. A similar trend was shown 48 h after the experiments’ establishment (Table 3), confirming once more the high nematicidal activity of CuFe NPs. Finally, increasing the exposure period to 48 or 72 h resulted in same EC50 values (70 and 72 μg/mL 72 h after M. incognita J2 immersion to Cu and CuFeO2 NPs, respectively, Table 3). This fact demonstrates that all J2 found immotile after 24 h of immersion to test solutions had been dead and did not regain their activity later. The paralysis activity of the tested NPs against M. javanica was found similar (Table 3) and the EC50 values of Cu, CuFe and CuFeO2 NPs, after 24 h immersion of M. javanica J2 in test solutions, were calculated at 65, 15 and 75 μg/mL, respectively. Again, the activity of all tested NPs was retained after 72 h of exposure. Natural death in control for M. incognita and M. javanica did not exceed 5% for all assessment dates. In all cases, J2 found immotile after 72 h of exposure were recorded as dead because they did not regain their motility after moving to water. For comparison, copper sulfate and fosthiazate were used as chemical control, with estimated EC50/24h of 32.9 and 0.79 μg/mL, or 24.5 and 0.89 μg/mL for M. incognita or M. javanica J2, respectively.
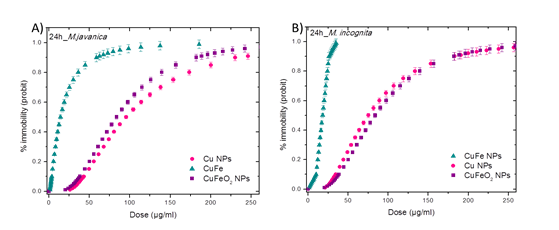
Figure 4: Dose response curves of (A) M. javanica and (B) M. incognita nematodes treated with Cu, CuFe and CuFeO2 NPs solutions for 24 h. Each point represents the average percent number of paralyzed nematodes of five replicates per treatment (× two experiments replications) after elimination of natural death counts in the control.
Sample |
EC50 (μg/mL), 24 h |
EC50 (μg/mL), 48 h |
EC50 (μg/mL), 72 h |
|||
M. incognita |
M. javanica |
M. incognita |
M. javanica |
M. incognita |
M. javanica |
|
Cu |
75 ± 5 |
65 ± 4 |
72 ± 2 |
63 ± 5 |
70 ± 3 |
61 ± 2 |
CuFe |
18 ± 3 |
15 ± 2 |
9 ± 2 |
13 ± 2 |
-* |
-* |
CuFeO2 |
73 ± 4 |
75 ± 3 |
73 ± 2 |
74 ± 4 |
72 ± 4 |
72 ± 3 |
*100% paralyzed at the lowest concentration dose.
Table 3: Estimated EC50 values for Cu, CuFe and CuFeO2 NPs calculated after 24, 48 and 72 h of M. incognita and M. javanica J2 immersion in test solutions and expressed as EC50 ± SD (μg/mL).
3.4 Effect of NPs on the nematode’s life cycle
Cu, CuFe and CuFeO2 NPs were evaluated in vivo in pot experiments (Table 4) against M. incognita and clear dose response relationships were established based on the numbers of females and galls/g of root tissues. The EC50 values corresponding to the reduction of females/ g of root tissues for CuFe, Cu and CuFeO2 NPs, were calculated at 0.03, 0.05 and 0.2 μg/g soil respectively (Table 4). Similar EC50 values were found considering the reduction of galls/g of root tissues (data not shown). Fosthiazate tested at the registered dose of 2 μg ai g/soil resulted 100% efficacy. Interestingly, a significant increase was observed in the fresh shoot and root weight of tomato plants treated at the three higher doses of CuFe NPs (Table 5) (Figure 5). In all cases, no phytotoxicity was observed in the tomato plants after treatment with metal composts. The infestation in control plants was 80 females/g of root tissues.
Sample |
Females/g root reduction |
|
EC50 (μg/g soil) |
SD |
|
Cu |
0.05 |
0.01 |
CuFe |
0.03 |
0.01 |
CuFeO2 |
0.20 |
0.02 |
Table 4: EC50 values as calculated in pot experiments following M. incognita exposure to soils amended with Cu, CuFe and CuFeO2 NPs, expressed as EC50 ± SD (μg/g soil).
Treatment μg/g soil |
Fresh shoot weight* (g) |
Fresh root weight* (g) |
||
Cu NPs |
CuFe NPs |
Cu NPs |
CuFe NPs |
|
0 (Control) |
5.050b |
5.050b |
1.532b |
1.532a |
0.2 |
5.286b |
6.172d |
1.540b |
1.647b |
0.1 |
5.474c |
5.746c |
1.498b |
1.612b |
0.05 |
5.188bc |
5.344bc |
1.526b |
1.530a |
0.025 |
4.538a |
4.710a |
1.366a |
1.516a |
*Data are presented as means of ?ve replicates with standard deviations. Means followed by the same letter in the same column are not signi?cantly different according to Tukey’s test (P ≤ 0.05).
Table 5: Growth parameters (fresh shoot and root weight) of tomato plants (Solanum lycopersicum) in soil infested with M. incognita nematodes and containing the different rates of Cu and CuFe NPs.
Figure 5: Tomato plants and their roots treated with Cu, CuFe and CuFeO2 NPs at the dose of 0.2 μg g/soil in comparison to the untreated-control plants.
4. Discussion
The root-knot nematodes, Meloidogyne spp. are difficult to control and the agricultural sector needs green and effective nematicides towards that direction. Nanotechnology could offer novel approaches for the solution of this problem since the performances of conventional pesticide formulations are often ameliorated through the construction of nanotechnology [34, 35]. A plethora of chemical, physical and biological techniques continues to evolve leading to the production of noble metal nanoparticles, some of whom are of use against nematodes [36]. Recent research on silver nanoparticles (Ag-NP), “naked” or of botanical products, showed significant nematicidal effect against M. incognita [37, 38]. In that vein, different compositions of Cu/Fe NPs were synthesized in the presence of the biocompatible surfactant PEG8000 and evaluated as nematicidals for the ?rst time. Two nematode species were investigated, namely M. incognita and M. javanica, and similar EC50 values were established. CuFe NPs was the best effective product in paralyzing M. incognita, with an EC50/24h value of 18 μg/mL.
The nematicidal activity of the NPs was determined in the descending order of CuFe (Cu0) > Cu (Cu0) = CuFeO2 (Cu1+), with the last two compositions revealing similar efficacy. Copper salts have already been reported of nematicidal activity when used in combination with maleimide derivatives [39], while metal complexes have been found effective both against the root-knot nematodes and the soil born fungi Fusarium oxysporum [40]. In specific copper sulphate, principally used as a fungicide but also for treating copper-deficient soils, has been reported to exhibit an EC50 value of 280 μg/mL against M. javanica [41] while Eloh et al. [39] reported an EC50 value of 48.6 μg/mL against M. incognita. Also, application of a mixture of maleic acid and copper sulfate reduced RKN disease on tomato by 51.72% in a pot experiment, suppressed gall formation on melon and decreased nematode population density in the soil [42]. Regarding copper based NPs, in previous studies, against plant pathogenic bacteria, among NPs of the same sizes with varyin compositions such as Cu (Cu0), Cu2O (Cu1+) and CuO (Cu2+) NPs, metallic copper has been found the most potent [14].
In this study the CuFe NPs exhibited the highest activity, but we can’t overlook the fact that in the case of CuFe NPs a higher ionic release occurs, after 24 h dispersion in water, than in the case of Cu NPs (19.8 μg/ml and 1.6 μg/mL, respectively). Considering that the nematicidal activity of copper sulfate has been determined at EC50/24h = 32.9 μg/mL, the potent activity of CuFe NPs could partially be attributed to copper ions released 24 hours after dispersion of CuFe NPs in water. On the contrary iron has not significant antimicrobial properties [43], while the nematicidal activity of iron sulfate against M. incognita has been reported at 126 ± 48 μg/mL [39], much higher than the concentration of free iron (0.5 μg/mL) released after 24 h of dispersion and therefore the effect of copper/iron based NPs on Meloidogyne spp. has further to be studied.
Furthermore, Cu, CuFe and CuFeO2 NPs arrest the biological cycle of M. incognita in tomato roots when used in pot experiments. Among the tested compositions, CuFe NPs revealed once again the highest efficacy and the lowest EC50=0.03 μg/g soil. Finally, the nematicidal activity followed the same descending order of CuFe(Cu0) > Cu(Cu0) > CuFeO2(Cu1+). The fact that no phytoxicity was observed in tomato plants treated with the tested compositions, while the bimetallic CuFe NPs resulted in significant increase of fresh shoot and root weight in the three higher doses, indicate an additional beneficial role (Figure 5) (Table 5) and should be considered together with the efficacy parameters in case it would be addressed as a pest management tool.
On the other hand, both copper and iron are essential trace elements for plants’ growth [44] participating in basic photosynthesis processes and bio functions of plants at the cellular level; while their lack may lead to structural damages [43]. Although recent published results report on root exposure of plants to NPs, the plant root uptake of NPs is still controversial [47]. Recent studies on Fe NPs revealed the fate of iron in soil being associated with its additive form. Coated NPs, as in the present study, were proved to alter the surface chemistry and interaction with the environment [45].Specifically pegylation has been suggested to insert stealth characteristics to NPs and promote the biofunctions in comparison to the uncoated NPs [46].Hydrodynamic sizes of the NPs were found under 260 nm (Figure 1S-Supplementary file) indicating aggregation effects. The particle size of NPs is one of the most important properties impacting their plant root uptake, although published results are contradictory[47].NPs with sizes up to 3 μ have been reported to transport in plant tissues from root to shoot through the apoplastic pathway [47].
However, when NPs are applied to soil, usually biotic and abiotic transformations such as redox reactions, aggregation and dissolution of NPs occur, resulting in changes in their bioavailability, toxicity and fate of the NPs. Thus, NPs exposed to plants in the soil may retain different properties from their original ones. Therefore, it is possible their transformation into bioavailable ionic forms such as Cu2+/Cu1+ and Fe2+/Fe3+ and further transportation in the plant tissues. The fact that only CuFe NPs were found to enhance growth parameters might be attributed to a combined effect of the two elements. Plants evaluate iron in the ionic forms of Fe2+and Fe3+, but only Fe2+ stimulates growth parameters [43]. In fact, in case of CuFeO2 where iron participates as Fe3+ there is no evidence of growth enhancement. Considering CuFe NPs, we assume that the metallic form of iron transforms to the ionic bioavailable Fe2+; although the combination with soil micronutrients cannot be excluded. This is in agreement with previous studies, reporting treatments of metallic Cu combined with metallic Fe NPs on wheat seeds resulting in an increase of sugar content [48]. Conclusively, copper/iron based NPs were found promising to control nematodes while CuFe NPs can be further suggested as plant growth enhancers.
Acknowledgments
Special thanks are due to T. Koufakis and AGRIS SA for providing seeds and seedlings, and to E. A. Tzortzakakis of Hellenic Agricultural Organization-Demeter, Athens (ELGO) for providing Meloidogyne incognita and Meloidogyne javanica.
References
- Back MA, Haydock PPJ, Jenkinson P. Disease complexes involving plant parasitic nematodes and soilborne pathogens. Plant Pathology 51 (2002): 683-697.
- Singh S, Singh B, Singh AP. Nematodes: A Threat to Sustainability of Agriculture. Proc Environ Sci 29 (2015): 215-216.
- Ntalli NG, Ferrari F, Giannakou I, et al. Synergistic and antagonistic interactions of terpenes against Meloidogyne incognita and the nematicidal activity of essential oils from seven plants indigenous to Greece. Pest Manag Sci 67 (2011): 341-351.
- Chitwood DJ. Phytochemical based strategies for nematode control. Annu Rev Phytopathology 40 (2002): 221-249.
- Marschner H, Marslin G, Sheeba CJ, et al. Mineral nutrition of higher plants. Front Plant. Sci 8 (2017): 832.
- Yruela I. Copper in plants: acquisition, transport and interactions. Funct Plant Biol 36 (2009): 409-430.
- Lav R, Khot SS, Joe MM, et al. Applications of nanomaterials in agricultural production and crop protection: A review. Crop Protection 35 (2012): 64-70.
- Fortunati E, Mazzaglia A, Balestra GM. Sustainable control strategies for plant protection and food packaging sectors by natural substances and novel nanotechnological approaches. J Sci Food Agric 99 (2019): 986-1000.
- Ali A, Hira Zafar, MZ Ul, et al. Synthesis, characterization, applications, and challenges of iron oxide nanoparticles. Nanotechnol Sci Appl 9 (2016): 49.
- Martin-Fernandez C, Solti Á, Czech V, et al. Response of soybean plants to the application of synthetic and biodegradable Fe chelates and Fe complexes. Plant PhysiolBiochem 118 (2017): 579-588.
- Arakha M, Pal S, Samantarrai D, et al. Antimicrobial activity of iron oxide nanoparticle upon modulation of nanoparticle-bacteria interface. Scientific Reports 5 (2015): 14813.
- He S, Feng Y, Ren H, et al. The impact of iron oxide magnetic nanoparticles on the soil bacterial community. J Soils Sediments 11 (2011): 1408-1417.
- Rui M, Ma C, Hao Y, et al. Iron Oxide Nanoparticles as a Potential Iron Fertilizer for Peanut (Arachishypogaea) Front Plant Sci 7 (2016): 815.
- Jeyasubramanian K, Thoppey UUG, Hikku GS, et al. Enhancement in growth rate and productivity of spinach grown in hydroponics with iron oxide nanoparticles. RSC Advances 6 (2016): 15451-15459.
- Ingle AP, Duran N, Rai M. Bioactivity, mechanism of action, and cytotoxicity of copper-based nanoparticles: a review. Appl Microbiol Biotechnol 98 (2014): 1001-1009.
- Giannousi K, Pantazaki A, Dendrinou-Samara A. Copper-Based Nanoparticles as Antimicrobials. Nanostr For Antimic Therapy 23 (2017): 515-529.
- Lux Research Stealth Success, Nanomaterials State of the Market Q3 2008: Stealth Success, Broad Impact. Broad Impact Report (2008).
- Mishra S, Keswani C, Abhilash PC, et al. Integrated Approach of Agri-nanotechnology: Challenges and Future Trends. Front Plant Sci 8 (2017): 471.
- Rastogi A, Zivcak M, Sytar O, et al. Impact of Metal and Metal Oxide Nanoparticles on Plant: A Critical Review. Fron in chem 5 (2017): 78.
- Adams J, Wright M, Wagner H, et al. Cu from dissolution of CuO nanoparticles signals changes in root morphology. Plant Physiol. Biochem 110 (2017): 108-117.
- Lee WM, An YJ, Yoon H, et al. Toxicity and bioavailability of copper nanoparticles to the terrestrial plants mung bean (Phaseolus radiatus) and wheat (Triticum aestivum): plant agar test for water-insoluble nanoparticles. Environ Toxicol Chem 27 (2008): 1915-1921.
- Ardakani AS. Toxicity of silver, titanium and silicon nanoparticles on the root-knot nematode, Meloidogyne incognita, and growth parameters of tomato. Nematology 15 (2013): 671-677.
- Cromwell WA, Yang J, Starr JL, et al. Nematicidal Effects of Silver Nanoparticles on Root-knot Nematode in Bermudagrass. J Nematol 46 (2014): 261-266.
- Antonoglou O, Giannousi K, Arvanitidis J, et al. Elucidation of one step synthesis of PEGylated CuFe bimetallic nanoparticles. Antimicrobial activity of CuFe@PEG vs Cu@PEG J of Inorg Biochem 177 (2017): 159-170.
- Salmaso S, Caliceti P. Stealth Properties to Improve Therapeutic Efficacy of Drug Nanocarriers. J Drug Deliv (2013): 19.
- Gkanatsiou Ch, Karamanoli Κ, Menkissoglu-Spiroudi U, et al. Composition effect of Cu-based nanoparticles on phytopathogenic bacteria: Antibacterial studies and phytotoxicity evaluation. Polyhedron 170 (2019): 395-403.
- Antonoglou O, Pantazaki A, Mourdikoudis S, et al. Biological relevance of CuFeO2 nanoparticles: Antibacterial and anti-inflammatory activity, genotoxicity, DNA and protein interactions. Materials Science and Engineering C 99 (2019): 264-274.
- Hussey RS, Barker KR. A comparison of methods of collecting inocula of Meloidogyne spp., including a new technique. Plant Dis Rep 57 (1973): 1025-1028.
- Ntalli NG, Oplos Ch, Michailidis M, et al. Strong synergistic activity and egg hatch inhibition by (E,E)-2,4-decadienal and (E)-2-decenal in Meloidogyne species. J Pest Sci 89 (2016): 565-579.
- Pantelelis I, Karpouzas DG, Menkissoglu-Spiroudi U, et al. Influence of soil physicochemical and biological properties on the degradation and adsorption of the nematicide fosthiazate. J Agric Food Chem 54 (2006): 6783-6789.
- Byrd DW, Krikpatrick T, Barker KR. An improved technique for cleaning and staining plant tissue for detection of nematodes. J of Nematology 15 (1983): 142-143.
- Oplos C, Eloh K, Menkissoglu-Spiroudi U, et al. Nematicidal Weeds, Solanum nigrum and Datura stramonium. Journal of Nematology 50 (2018): 3.
- Puntener W. Manual for Field Trials in Plant Protection. Ciba-Geigy Limited (1981): 205.
- Khot LR, Sankaran S, Maja JM, et al. Applications of nanomaterials in agricultural production and crop protection: A review. Crop Prot 35 (2012): 64-70.
- De Oliveira JL, Campos EV, Bakshi M, et al. Application of nanotechnology for the encapsulation of botanical insecticides for sustainable agriculture: prospects and promises. Biotechnol. Adv 32 (2014): 1550-1561.
- Bhau BS, Phukon P, Ahmed R, et al. Microbial Inoculants in Sustainable Agricultural Productivity. Functional Applications 2 (2016): 253-270.
- Abbassy MA, Abdel-Rasoul MA, Atef Nassar MK, et al. Nematicidal activity of silver nanoparticles of botanical products against root-knot nematode, Meloidogyne incognita. Archives of Phytopathology and Plant Protection 50 (2017): 17-18, 909-926.
- Abdellatif KF, Abdelfattah RH, El- Ansary MSM. Green Nanoparticles Engineering on Root-knot Nematode Infecting Eggplants and Their Effect on Plant DNA Modification. Iran J Biotechnol 14 (2016): 250-259.
- Eloh K, Demurtas M, Mura MG, et al. Potent Nematicidal Activity of Maleimide Derivatives on Meloidogyne incognita. J Agric Food Chem 64 (2016): 4876-4881.
- Nawaz M, Abbasi MW, Hisaindee S, et al. Synthesis, spectral studies and biological evaluation of 2-aminonicotinic acid metal complexes. Spectrochimica Acta-Part A: Molecular and Biomolecular Spectr 161 (2016): 39-43.
- Rahul S, Chandrashekhar P, Hemant B, et al. Nematicidal activity of microbial pigment from Serratia marcescens. Natural Product Research 28 (2014): 1399-1404.
- Yeon J, Park AR, Kim YJ, et al. Control of root-knot nematodes by a mixture of maleic acid and copper sulfate. Applied Soil Ecology 141 (2019): 61-68.
- Yuan J, Chen Y, Li H, et al. New insights into the cellular responses to iron nanoparticles in Capsicum annuum. Sci Rep 8 (2018): 3228.
- Bindraban S, Dimkpa C, Nagarajan L, et al. Revisiting fertilisers and fertilisation strategies for improved nutrient uptake by plants. Biology and Fertility of Soils 51 (2015): 897-911.
- Chanteau B, Frsnais J, Berret JF. Electrosteric enhanced stability of functional sub-10 nm cerium and iron oxide particles in cell culture medium. Langmuir 25 (2009): 9064-9070.
- Barrios AC, Rico CM, Trujillo-Reyes J, et al. Effects of uncoated and citric acid coated cerium oxide nanoparticles, bulk cerium oxide, cerium acetate, and citric acid on tomato plants. Sci Total Environ (2016): 563-564, 956-64.
- Lv J, Christie P, Zhang S. Uptake, translocation, and transformation of metal-based nanoparticles in plants: recent advances and methodological challenges. Environ Sci: Nano 6 (2019): 41-59.
- Yruela I. Copper in plants: acquisition, transport and interactions. Funct Plant Biol 36 (2009): 409-430.