Effect of Melatonin on Erythrocyte Deformability in Mice With Ischemia-Reperfusion Injury in Skeletal Muscle
Article Information
Hakan Kartal*, Faruk Metin Comu
Department of Cardiovascular Surgery, Gulhane Education and Research Hospital, Ankara, Turkey
*Corresponding Authors: Hakan Kartal, Department of Cardiovascular Surgery, Gulhane Education and Research Hospital, Ankara, Turkey
Received: 27 July 2020; Accepted: 03 August 2020; Published: 17 August 2020
Citation: Hakan Kartal, Faruk Metin Comu. Effect of Melatonin on Erythrocyte Deformability in Mice With Ischemia-Reperfusion Injury in Skeletal Muscle. Journal of Surgery and Research 4 (2020): 262-271.
View / Download Pdf Share at FacebookAbstract
Aim: Erythrocyte deformability is the deformation movement that erythrocytes perform while passing through the capillary vessel in the microvascular area. Erythrocyte deformability is vital in microvascular circulation. The aim of this study was to investigate how melatonin affects the ability of erythrocytes to deform in mice who underwent ischemia-reperfusion in the lower limb.
Materials and methods: In the study, 30 male Swiss breed albino mice weighing 45-65 g were used. Mice were randomly divided into 5 groups, (Control group (C), melatonin control group (M), Dimethyl Sulfoxide (DMSO) group, ischemia-reperfusion (IR) group, melatonin - IR group (M+IR)), 6 being in each group. All groups were administered 100 IU/kg intravenous heparin bolus 30 minutes before the procedure. Lower limb ischemia (2 hours) and reperfusion (2 hours) protocols were applied to the IR and M+IR groups by clamping the main femoral artery. Melatonin was administered to M and M+IR groups 1 hour before the experimental procedure (10mg / kg intraperitoneal). After the experiment protocol mice were sacrificed by the intracardiac blood collection method. From the blood samples taken, erythrocytes were extracted from heparinized whole blood samples. Erythrocytes were passed through the filtration system and pressure changes were measured to investigate the erythrocyte deformation index.
Results: Control group, melatonin group, and DMSO group's deformability levels are observed to be at levels lower than or similar to melatonin group + IR and ischemia-reperfusion group. In addition, melatonin group + IR was found to be at a lower level than ischemia-reperfusion group in terms of deformability.
Conclusion: Unless there is an event having adverse effects on erythrocytes, melatonin has no effect on the deformability index. However, it is observed that mel
Keywords
Melatonin; Ischemia-reperfusion injury; Erythrocyte deformability
Melatonin articles, Ischemia-reperfusion injury articles, Erythrocyte deformability articles
Melatonin articles Melatonin Research articles Melatonin review articles Melatonin PubMed articles Melatonin PubMed Central articles Melatonin 2023 articles Melatonin 2024 articles Melatonin Scopus articles Melatonin impact factor journals Melatonin Scopus journals Melatonin PubMed journals Melatonin medical journals Melatonin free journals Melatonin best journals Melatonin top journals Melatonin free medical journals Melatonin famous journals Melatonin Google Scholar indexed journals Ischemia-reperfusion injury articles Ischemia-reperfusion injury Research articles Ischemia-reperfusion injury review articles Ischemia-reperfusion injury PubMed articles Ischemia-reperfusion injury PubMed Central articles Ischemia-reperfusion injury 2023 articles Ischemia-reperfusion injury 2024 articles Ischemia-reperfusion injury Scopus articles Ischemia-reperfusion injury impact factor journals Ischemia-reperfusion injury Scopus journals Ischemia-reperfusion injury PubMed journals Ischemia-reperfusion injury medical journals Ischemia-reperfusion injury free journals Ischemia-reperfusion injury best journals Ischemia-reperfusion injury top journals Ischemia-reperfusion injury free medical journals Ischemia-reperfusion injury famous journals Ischemia-reperfusion injury Google Scholar indexed journals Erythrocyte deformability articles Erythrocyte deformability Research articles Erythrocyte deformability review articles Erythrocyte deformability PubMed articles Erythrocyte deformability PubMed Central articles Erythrocyte deformability 2023 articles Erythrocyte deformability 2024 articles Erythrocyte deformability Scopus articles Erythrocyte deformability impact factor journals Erythrocyte deformability Scopus journals Erythrocyte deformability PubMed journals Erythrocyte deformability medical journals Erythrocyte deformability free journals Erythrocyte deformability best journals Erythrocyte deformability top journals Erythrocyte deformability free medical journals Erythrocyte deformability famous journals Erythrocyte deformability Google Scholar indexed journals Melatonin articles Melatonin Research articles Melatonin review articles Melatonin PubMed articles Melatonin PubMed Central articles Melatonin 2023 articles Melatonin 2024 articles Melatonin Scopus articles Melatonin impact factor journals Melatonin Scopus journals Melatonin PubMed journals Melatonin medical journals Melatonin free journals Melatonin best journals Melatonin top journals Melatonin free medical journals Melatonin famous journals Melatonin Google Scholar indexed journals Skeletal Muscle articles Skeletal Muscle Research articles Skeletal Muscle review articles Skeletal Muscle PubMed articles Skeletal Muscle PubMed Central articles Skeletal Muscle 2023 articles Skeletal Muscle 2024 articles Skeletal Muscle Scopus articles Skeletal Muscle impact factor journals Skeletal Muscle Scopus journals Skeletal Muscle PubMed journals Skeletal Muscle medical journals Skeletal Muscle free journals Skeletal Muscle best journals Skeletal Muscle top journals Skeletal Muscle free medical journals Skeletal Muscle famous journals Skeletal Muscle Google Scholar indexed journals microvascular circulation articles microvascular circulation Research articles microvascular circulation review articles microvascular circulation PubMed articles microvascular circulation PubMed Central articles microvascular circulation 2023 articles microvascular circulation 2024 articles microvascular circulation Scopus articles microvascular circulation impact factor journals microvascular circulation Scopus journals microvascular circulation PubMed journals microvascular circulation medical journals microvascular circulation free journals microvascular circulation best journals microvascular circulation top journals microvascular circulation free medical journals microvascular circulation famous journals microvascular circulation Google Scholar indexed journals red blood cell deformability articles red blood cell deformability Research articles red blood cell deformability review articles red blood cell deformability PubMed articles red blood cell deformability PubMed Central articles red blood cell deformability 2023 articles red blood cell deformability 2024 articles red blood cell deformability Scopus articles red blood cell deformability impact factor journals red blood cell deformability Scopus journals red blood cell deformability PubMed journals red blood cell deformability medical journals red blood cell deformability free journals red blood cell deformability best journals red blood cell deformability top journals red blood cell deformability free medical journals red blood cell deformability famous journals red blood cell deformability Google Scholar indexed journals intramuscular injection articles intramuscular injection Research articles intramuscular injection review articles intramuscular injection PubMed articles intramuscular injection PubMed Central articles intramuscular injection 2023 articles intramuscular injection 2024 articles intramuscular injection Scopus articles intramuscular injection impact factor journals intramuscular injection Scopus journals intramuscular injection PubMed journals intramuscular injection medical journals intramuscular injection free journals intramuscular injection best journals intramuscular injection top journals intramuscular injection free medical journals intramuscular injection famous journals intramuscular injection Google Scholar indexed journals intracardiac blood articles intracardiac blood Research articles intracardiac blood review articles intracardiac blood PubMed articles intracardiac blood PubMed Central articles intracardiac blood 2023 articles intracardiac blood 2024 articles intracardiac blood Scopus articles intracardiac blood impact factor journals intracardiac blood Scopus journals intracardiac blood PubMed journals intracardiac blood medical journals intracardiac blood free journals intracardiac blood best journals intracardiac blood top journals intracardiac blood free medical journals intracardiac blood famous journals intracardiac blood Google Scholar indexed journals
Article Details
1. Introduction
Acute limb ischemia is defined as a sudden decrease in limb perfusion that potentially threatens the viability of the limb [1]. Blood reperfusion is required to restore the metabolic function of ischemic tissue; however, the reperfusion process often causes increased reactive oxygen species (ROS) and neutrophil infiltration and also induces inflammatory reactions that can cause local and distant tissue damage [2]. IschemIa reperfusIon damage Is not only IschemIc tIssue; It damages blood [3], heart [4], lungs [5] and many tissues.
The first target of ischemia and subsequent reperfusion injury is microvascular circulation [6]. All these processes that occur during or after ischemia-reperfusion can cause changes in red blood cell deformability [7].
The ability of the erythrocytes to deform is the ability to change the shape and return to the previous form. The ability of erythrocytes (about 7.2 μm in diameter) to pass through capillaries as small as 3-4 μm in diameter is one of the most important determinants for erythrocytes' survival in circulation. This is also very important for microcirculatory flow and to supply enough oxygen to the tissues [8].
Melatonin (N-acetyl-5-methoxytryptamine) is an endogenous hormone produced mainly by the pineal gland in the brain [9]. Since erythrocytes are in close contact with all hormones, including melatonin, it is estimated that melatonin may affect the functions of erythrocytes, such as deformability [10].
2. Materials and Methods
2.1 Animals and chemicals
The study was conducted at Gazi University Laboratory Animal Breeding and Experimental Researches Center (GÜDAM) with the permission obtained from Gazi University Animal Experiments Local Ethics Committee with the code number G.Ü.ET-19.047. Melatonin used in the study was provided from Sigma-Aldrich company without any institution or company support. Blood samples were analyzed in the physiology laboratory of KIrIkkale University. All procedures were carried out in accordance with the Standards for the Care and Use of Laboratory Animals.
In the study, 30 male Swiss breed albino mice weighing 45-65 g were used. Mice were randomly divided into 5 groups (Control group (C), melatonin control group (M), Dimethyl Sulfoxide group (DMSO), ischemia-reperfusion (IR) group, melatonin + ischemia-reperfusion group (M + IR)), 6 being in each group.
2.2 Technical procedure
At the beginning of the experiment, anesthesia was achieved by intramuscular injection by administering 50 mg/kg dose of ketamine hydrochloride (Ketalar® vial, Parke-Davis, USA) and 10 mg/kg xylazine hydrochloride (Alfazyne, 2%, Ege Vet). The procedure was performed under a heat lamp with the mice in the supine position. Anticoagulation with heparin was applied intraperitoneally to all groups (100 u/ kg). In order for heparin not to cause differentiation in the study results, the same amount was also applied intraperitoneally to the groups without ischemia-reperfusion. A longitudinal skin incision was performed on the inguinal region. Common-superficial and profundal femoral artery were explored. An atraumatic microvascular clamp was placed in the common femoral artery. Following the 120-minute ischemic period, the microvascular clamp on the common femoral artery was removed and reperfusion was achieved for 120 minutes. The same time (240 minutes) was awaited by applying an inguinal incision on the mice forming the control group, but IR was not generated in these groups. Mice were sacrificed by intracardiac blood collection method.
Control group group (Gr C, n = 6): After heparin application, only an inguinal incision was performed and closed without ischemia in this group of mice, and four hours after the procedure, intracardiac blood samples were taken from the mice under anesthesia.
Melatonin group group (Gr M, n = 6): In this group, 10 mg/kg dose of melatonin was administered intraperitoneally, without ischemia. Only an inguinal incision was performed and closed. Four hours after the procedure, intracardiac blood samples were taken from the mice under anesthesia.
DMSO group group (Gr DMSO, n = 6): After heparin administration, DMSO as much as the DMSO amount given to the melatonin groups (50 mg melatonin dissolved in 1 ml DMSO) was administered intraperitoneally without ischemia to this group of mice in order to examine the effects of DMSO, in which melatonin was dissolved, on erythrocytes. After that only inguinal incision was made and closed without ischemia. Four hours after the procedure, intracardiac blood samples were taken from the mice under anesthesia.
Ischemia-reperfusion group group (Gr IR, n = 6): After heparin administration, an inguinal incision was made, without melatonin injection, on the mice in this group. Atraumatic microvascular clamp was placed on the main femoral artery, and after 120 minutes of ischemia and 120 minutes of reperfusion, intracardiac blood samples were taken from the mice under anesthesia.
Melatonin ischemia-reperfusion group group (Gr M+IR, n = 6): After heparin administration, melatonin was administered intraperitoneally at a dose of 10 mg/kg 1 hour before application of ischemia in this group of mice. An inguinal incision was performed. Atraumatic microvascular clamp was placed on the main femoral artery, and after 120 minutes of ischemia and 120 minutes of reperfusion, intracardiac blood samples were taken from the mice under anesthesia.
2.3 Deformability measurements
Erythrocyte deformability indexes were determined in KIrIkkale University Faculty of Medicine Physiology Laboratory, which is isolated against external factors such as heat and electricity. A constant flow filtrometer system was used to measure erythrocyte deformability. In the system, calibration was achieved before use in the pressure ranges 0.5-4 cmH2O. Within 2 hours, measurement procedures were started in the blood samples taken. Erythrocyte packages were formed by washing the heparinized blood 3 times in PBS (Phosphate-buffered saline) buffer. Then, erythrocytes brought to room temperature (25°C) were resuspended in PBS buffer as Htc: 5% and 10ml samples were prepared. Nucleopore polycarbonate filters (Nuclepore Polycarbonate filter, Merck-Whatman) with a diameter of 25mm and a pore size of 5 μm were used in the system. Separate filters were used for each study. 1.5 ml/min. constant flow rate (infusion pump, Biopac Systems Inc. - Commat, Ltd.) was achieved, and the resulting filtration pressure was measured in cmH 2 O. Actual pressure changes were monitored by being transferred to the computer via Data Acquisition System (Data Acquisition System Biopac systems Inc. USA). Two measurements were made for each sample and the mean was used. Many variables such as measured pressure values and time to reach that level were recorded and analyzed.
P L: Erythrocyte Suspension Filtration Pressure
P T: Buffer Solution Filtration Pressure
Relative pressure values were calculated; Rrel: P Erythrocyte /P Buffer
The relative pressure values obtained give us insight about the deformability of erythrocytes. An increase in that value indicates a negative effect on erythrocyte deformability.
2.4 Statistical method
Shapiro-Wilk test was performed to examine the normality levels of deformability measurements. According to the test result, since the distribution of the deformability measurement was not parametric and the sample numbers within the groups were low, analyses were made with non-parametric test approaches. In the analysis of the data, descriptive statistics are presented with mean, standard deviation values. For the study groups, the Mann- Whitney U test was used. In the study, p values less than 0.05 were considered statistically significant. All analyses were made using SPSS 22.0 software package.
3. Results
In our study, there was no difference in terms of deformability index between the Control group and the Melatonin group (p = 0.95). There was no difference in terms of the deformability index between Control group and DMSO group (p = 0.95). Deformability index levels were different between control group and ischemia-reperfusion group, and ischemia-reperfusion group had higher deformability index levels (p = 0.01). In addition, deformability index levels were different between control group and melatonin group + IR, and melatonin group + IR had higher deformability index levels (p = 0.01).
In the study, there was no difference between the melatonin group and the DMSO group in terms of the deformability index (p = 0.97). Deformability index levels were different between melatonin group and ischemia-reperfusion group, ischemia-reperfusion group had higher deformability index levels (p = 0.01). In the study, deformability index levels were different between melatonin group and melatonin group + IR, and melatonin group + IR had higher deformability index levels (p = 0.01).
Deformability index |
Group Control X±s.s 1,76±0,19 |
Group Melatonin |
p |
X±s.s |
|||
1,78±0,19 |
0,95 |
||
Group DMSO |
p |
||
X±s.s |
|||
1,79±0,19 |
0,91 |
||
Group Ischemia Reperfusion |
p |
||
X±s.s |
|||
2,96±0,19 |
0,01* |
||
Group Melatonin + IR |
p |
||
X±s.s |
|||
2,21±0,22 |
0,01* |
** Mann-Whitney U test was made. * significant difference p <0.05
Table 1: Melatonin group, DMSO group, Ischemia-reperfusion group and Melatonin group + IR 's comparison with the Control group
Deformability index |
Group Melatonin X±s.s |
Group DMSO |
p |
X±s.s |
|||
1,79±0,19 |
0,97 |
||
Group Ischemia Reperfusion |
p |
||
X±s.s |
|||
2,96±0,19 |
0,01* |
||
Group Melatonin + IR |
p |
||
X±s.s |
|||
2,21±0,22 |
0,01* |
** Mann-Whitney U test was made. * significant difference p <0.05
Table 2: Comparison of DMSO group, ischemia-reperfusion group and melatonin group + IR 's group with melatonin group
Deformability index |
Group DMSO X±s.s 1,79±0,19 |
Group Ischemia Reperfusion |
p |
X±s.s |
|||
2,96±0,19 |
0,01 |
||
Group Melatonin+IR |
p |
||
X±s.s |
|||
2,21±0,22 |
0,01 |
** Mann-Whitney U test was made. * significant difference p <0.05
Table 3: Comparison of ischemia-reperfusion group and melatonin group + IR with DMSO group
In the study, deformability index levels were different between DMSO group and ischemia-reperfusion group, and ischemia-reperfusion group had higher deformability index levels (p = 0.01). Deformability index levels were different between DMSO group and melatonin group + IR, and melatonin group + IR had higher deformability index levels (p = 0.01).
Deformability index |
Group Ischemia Reperfusion |
Melatonin+IR |
p |
X±s.s |
X±s.s |
||
2,96±0,19 |
2,21±0,22 |
0,03* |
** Mann-Whitney U test was made. * significant difference p <0.05
Table 4: Comparison of ischemia-reperfusion group and melatonin group + IR
In the study, deformability index levels were different between melatonin group + IR and ischemia-reperfusion group, and ischemia-reperfusion group had higher deformability index levels (p = 0.01).
Generally, deformability index levels of the control group, melatonin group, and DMSO group were similar to each other and at lower levels than the melatonin group + IR and ischemia-reperfusion group. In addition, in terms of the deformability index, the melatonin group + IR was at a lower level than the ischemia-reperfusion group.
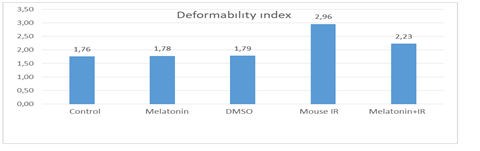
Figure 1: Study Groups and Deformability indexes
4. Discussion
The effects of the ischemia-reperfusion injury have been previously studied on many organs and tissues. It has been shown to affect many organs and tissues, including the brain, heart, liver, lung, kidney, skeletal muscles, testicular tissue, and endothelial tissue, and causes severe morbidity and mortality worldwide [11-18].
Impairment of the erythrocyte deformability feature may lead to increased blood viscosity, obstruction in microvessels, impaired perfusion, and ischemia. Although the underlying mechanisms are partially explained, ischemia-reperfusion injury affects blood and plasma viscosity, erythrocyte deformability, and aggregation [19,20]. Reduced deformability of erythrocytes leads to microcirculatory irregularities such as occlusion in the capillary vessels and decreased oxygenation for the whole body [21]. Therefore, the deformability feature of erythrocytes, which are the main effect area of ischemia-reperfusion injury and play the most important role in the microvascular bed, also play an important role in protecting against reperfusion injury.
The hormone melatonin, which acts according to circadian rhythm and is secreted by the pineal gland in the dark, plays a role in the regulation of many physiological functions in the body [22-24]. Endogenously produced melatonin provides a strong defense against ischemia-reperfusion injury as well as being protective against lipid peroxidation due to oxidative damage [25-28].
In our study, the deformability index of erythrocytes was observed to increase in the ischemia-reperfusion group compared to the control groups (control group, melatonin group, DMSO group). This is proof that ischemia-reperfusion affects erythrocytes negatively and disrupts microvascular circulation.
There was no significant difference between the melatonin group and the control group deformability indexes. In a previous study, it has been observed that the increase and decrease in the plasma levels, between day and night, of melatonin, which has a circadian rhythm, does not have any effect on the deformability index of erythrocytes [7]. However, in another study, it was shown that melatonin corrected the erythrocyte deformability, which had deteriorated due to oxidative stress [29]. When evaluated together with the results of our study, melatonin alone does not provide additional benefit unless there is a condition that impairs erythrocyte deformability.
The fact that the DMSO group has a similar deformability index compared to the control group shows that DMSO, the solvent of melatonin, does not negatively affect deformability. In addition, the deformability index in the ischemia-reperfusion group was significantly higher than the melatonin + IR group. This indicates that melatonin positively affects the erythrocyte deformability that deteriorates due to reperfusion injury.
Our study aiming to investigate these controversial effects of melatonin on erythrocytes, regarding which there is no consensus in previous studies, shows that if there is no event negatively affecting erythrocytes, melatonin does not affect deformability index, but indeed positively does so in cases negatively affecting erythrocyte deformability, such as ischemia-reperfusion injury.
More in vivo and clinical studies are needed in the future to better understand the effects of melatonin on erythrocytes.
Limitations
Statistical analysis showed us that more animals should be used in the study groups. We consider that keeping the number of animals higher in future studies may help overcome these statistical problems.
Competing interests
The authors declare that there is no conflict of interest that could be perceived as prejudicing the impartiality of the research reported.
Reference
- Norgren L, Hiatt WR, Dormandy JA, Nehler MR, Harris KA, Fowkes FG; TASC II Working Group. Inter-Society Consensus for the Management of Peripheral Arterial Disease (TASC II). J Vasc Surg 45 (2007): S5-67.
- Grace PA. Ischaemia-reperfusion injury. Br J Surg 81 (1994): 637-47.
- Arslan M, Comu FM, Isik B, Ozturk L, Kesimci E. Effect of dexmedetomidine on erythrocyte deformability during ischemia-reperfusion injury of liver in diabetic rats. Bratisl Lek Listy 113 (2012): 687-91.
- Geldi O, Kubat E, Ünal CS, Canbaz S. Acetaminophen Mitigates Myocardial Injury Induced by Lower Extremity Ischemia-Reperfusion in Rat Model. Braz J Cardiovasc Surg 33 (2018): 258-264.
- Tural K, Ozden O, Bilgi Z, Kubat E, Ermutlu CS, Merhan O, et al. The protective effect of betanin and copper on heart and lung in end-organ ischemia reperfusion injury. Bratisl Lek Listy 121 (2020): 211-217.
- Welbourn CR, Goldman G, Paterson IS, Valeri CR, Shepro D, Hechtman HB. Pathophysiology of ischaemia reperfusion injury: central role of the neutrophil. Br J Surg 78 (1991): 651-5.
- Nemeth N, Lesznyak T, Szokoly M, Furka I, Miko I. Allopurinol prevents erythrocyte deformability impairing but not the hematological alterations after limb ischemia-reperfusion in rats. J Invest Surg 19 (2006): 47-56.
- Schmid-Schönbein H. Microrheology of erythrocytes, blood viscosity, and the distribution of blood flow in the microcirculation. Int Rev Physiol 9 (1976): 1-62.
- Pandi-Perumal SR, Trakht I, Srinivasan V, Spence DW, Maestroni GJ, Zisapel N, et al. Physiological effects of melatonin: role of melatonin receptors and signal transduction pathways. Prog Neurobiol 85 (2008): 335-53.
- Vazan R, Plauterova K, Porubska G, Radosinska J. Changes in erythrocyte deformability during day and possible role of melatonin. Endocr Regul 52 (2018): 17-20.
- Yang Y, Jiang S, Dong Y, Fan C, Zhao L, Yang X, et al. Melatonin prevents cell death and mitochondrial dysfunction via a SIRT1-dependent mechanism during ischemic-stroke in mice. J Pineal Res 58 (2015): 61-70.
- Li T, Zhang Z, Kolwicz SC Jr, Abell L, Roe ND, Kim M, et al. Defective Branched-Chain Amino Acid Catabolism Disrupts Glucose Metabolism and Sensitizes the Heart to Ischemia-Reperfusion Injury. Cell Metab 25 (2017): 374-385.
- Büyük B & Karakoç E. Effects of thiopental in cold ischemia in liver transplantation: An experimental study. Journal of Surgery and Medicine3 (2019): 143-148.
- Yip HK, Chang YC, Wallace CG, Chang LT, Tsai TH, Chen YL, et al. Melatonin treatment improves adipose-derived mesenchymal stem cell therapy for acute lung ischemia-reperfusion injury. J Pineal Res 54 (2013): 207-21.
- Block H, Herter JM, Rossaint J, Stadtmann A, Kliche S, Lowell CA, et al. Crucial role of SLP-76 and ADAP for neutrophil recruitment in mouse kidney ischemia-reperfusion injury. J Exp Med 209 (2012): 407-21.
- Andreas M, Schmid AI, Keilani M, Doberer D, Bartko J, Crevenna, et. al. Effect of ischemic preconditioning in skeletal muscle measured by functional magnetic resonance imaging and spectroscopy: a randomized crossover trial. J Cardiovasc Magn Reson 13 (2011): 32.
- Dokmeci D, Kanter M, Inan M, Aydogdu N, Basaran UN, Yalcin O, et al. Protective effects of ibuprofen on testicular torsion/detorsion-induced ischemia/reperfusion injury in rats. Arch Toxicol 81 (2007): 655-63.
- Maessen MFH, van Mil ACCM, Straathof Y, Riksen NP, Rongen GAPJM, Hopman MTE, et al. Impact of lifelong exercise training on endothelial ischemia-reperfusion and ischemic preconditioning in humans. Am J Physiol Regul Integr Comp Physiol 312 (2017): R828-R834.
- Khairy K, & Howard J. Minimum-energy vesicle and cell shapes calculated using spherical harmonics parameterization. Soft Matter 7 (2011): 2138-2143.
- Fischer TM. Shape memory of human red blood cells. Biophys J 86 (2004): 3304-13.
- Baskurt OK, Meiselman HJ. Blood rheology and hemodynamics. Semin Thromb Hemost 29 (2003): 435-50.
- Baba K, Davidson AJ, Tosini G. Melatonin Entrains PER2::LUC Bioluminescence Circadian Rhythm in the Mouse Cornea. Invest Ophthalmol Vis Sci 56 (2015): 4753-8.
- Claustrat B, Leston J. Melatonin: Physiological effects in humans. Neurochirurgie 61 (2015): 77-84.
- Baltaci SB, Mogulkoc R, Baltaci AK, Emsen A, Artac H. The effect of zinc and melatonin supplementation on immunity parameters in breast cancer induced by DMBA in rats. Arch Physiol Biochem 124 (2018): 247-252.
- Ma Z, Xin Z, Di W, Yan X, Li X, Reiter RJ, et al. Melatonin and mitochondrial function during ischemia/reperfusion injury. Cell Mol Life Sci 74 (2017): 3989-3998.
- Mehaisen GM, Saeed AM, Gad A, Abass AO, Arafa M, El-Sayed A. Antioxidant Capacity of Melatonin on Preimplantation Development of Fresh and Vitrified Rabbit Embryos: Morphological and Molecular Aspects. PLoS One 10 (2015): e0139814.
- Bazrgar M, Goudarzi I, Lashkarbolouki T, Elahdadi Salmani M. Melatonin ameliorates oxidative damage induced by maternal lead exposure in rat pups. Physiol Behav 151 (2015): 178-88.
- Gurer-Orhan H, Suzen S. Melatonin, its metabolites and its synthetic analogs as multi-faceted compounds: antioxidant, prooxidant and inhibitor of bioactivation reactions. Curr Med Chem 22 (2015): 490-9.
- Aydogan S, Yerer MB, Yapislar H. In vitro effects of melatonin on the filtrability of erythrocytes in SNP-induced oxidative stress. Clin Hemorheol Microcirc 30 (2004): 317-22.