A Case Series of Floppy Neonates: A Chain is No Stronger than its Weakest Link
Article Information
Dr Adarsh E1, Dr Srinivas Mahesh Prasad2, Dr Sagethya A3, Dr Surabhi HS4*
1Professor and HOD, Department of Paediatric, Rajarajeshwari medical College and hospital, Bangalore, India
2Assistant Professor, Department of Nephrology, Rajarajeshwari medical College and hospital, Bangalore, India
3Junior Resident, Department of Paediatric, Rajarajeshwari medical College and hospital, Bangalore, India
4Assistant Professor and Neonatologist, Department of Paediatric, Rajarajeshwari medical College and hospital, Bangalore, India
*Corresponding Author: Dr Surabhi HS, Assistant Professor and Neonatologist, Department of Paediatric, Rajarajeshwari medical College and hospital, Bangalore, India
Received: 19 August 2022; Accepted: 23 August 2022; Published: 16 September 2022
Citation: Adarsh E, Srinivas Mahesh Prasad, Sagethya A, Surabhi HS. A Case Series of Floppy Neonates: A Chain is No Stronger than its Weakest Link. Archives of Clinical and Medical Case Reports 6 (2022): 630-635.
View / Download Pdf Share at FacebookAbstract
Floppy infant syndrome (FIS) can be caused by a variety of disorders. FIS categorised into four main groups, namely, the central nervous system, the spinal motor neuron, peripheral nerves, neuromuscular junction and the muscles. Neonates might appear with a variety of signs depending on the cause of hypotonia. The perinatal asphyxia is the most common cause of central hypotonia in newborns. Other causes of central hypotonia include brain malformations, chromosomal abnormalities and inborn errors of metabolism like Non-ketotic hyperglycaemia (NKH). Infancy has a 13% prevalence of neuronal dysmigration disorder, which causes hypotonia. Peripheral causes (30%) include spinal muscular atrophy, myasthenia gravis, drug/toxin exposure, hereditary neuropathies, muscular dystrophies, congenital/metabolic myopathies, and congenital myotonic dystrophies. We'll take a look at how central and peripheral hypotonia manifest themselves in neonates in this case series. This study involves five neonates who required resuscitation after delivery and NICU care due to respiratory distress, necessitating examination and evaluation in order to diagnose these conditions during the neonatal period. Though uncommon, a high index of suspicion is necessary for diagnosis and can help with antenatal counselling.
Floppy Infant articles; Myopathies articles; Non-Ketotic Hyperglycaemia articles; Neuronal Dysmigration articles; Spinal Muscular Atrophy articles
Neonates articles Neonates Research articles Neonates review articles Neonates PubMed articles Neonates PubMed Central articles Neonates 2023 articles Neonates 2024 articles Neonates Scopus articles Neonates impact factor journals Neonates Scopus journals Neonates PubMed journals Neonates medical journals Neonates free journals Neonates best journals Neonates top journals Neonates free medical journals Neonates famous journals Neonates Google Scholar indexed journals COVID-19 articles COVID-19 Research articles COVID-19 review articles COVID-19 PubMed articles COVID-19 PubMed Central articles COVID-19 2023 articles COVID-19 2024 articles COVID-19 Scopus articles COVID-19 impact factor journals COVID-19 Scopus journals COVID-19 PubMed journals COVID-19 medical journals COVID-19 free journals COVID-19 best journals COVID-19 top journals COVID-19 free medical journals COVID-19 famous journals COVID-19 Google Scholar indexed journals atrophy articles atrophy Research articles atrophy review articles atrophy PubMed articles atrophy PubMed Central articles atrophy 2023 articles atrophy 2024 articles atrophy Scopus articles atrophy impact factor journals atrophy Scopus journals atrophy PubMed journals atrophy medical journals atrophy free journals atrophy best journals atrophy top journals atrophy free medical journals atrophy famous journals atrophy Google Scholar indexed journals Immunology articles Immunology Research articles Immunology review articles Immunology PubMed articles Immunology PubMed Central articles Immunology 2023 articles Immunology 2024 articles Immunology Scopus articles Immunology impact factor journals Immunology Scopus journals Immunology PubMed journals Immunology medical journals Immunology free journals Immunology best journals Immunology top journals Immunology free medical journals Immunology famous journals Immunology Google Scholar indexed journals Immunotherapy articles Immunotherapy Research articles Immunotherapy review articles Immunotherapy PubMed articles Immunotherapy PubMed Central articles Immunotherapy 2023 articles Immunotherapy 2024 articles Immunotherapy Scopus articles Immunotherapy impact factor journals Immunotherapy Scopus journals Immunotherapy PubMed journals Immunotherapy medical journals Immunotherapy free journals Immunotherapy best journals Immunotherapy top journals Immunotherapy free medical journals Immunotherapy famous journals Immunotherapy Google Scholar indexed journals treatment articles treatment Research articles treatment review articles treatment PubMed articles treatment PubMed Central articles treatment 2023 articles treatment 2024 articles treatment Scopus articles treatment impact factor journals treatment Scopus journals treatment PubMed journals treatment medical journals treatment free journals treatment best journals treatment top journals treatment free medical journals treatment famous journals treatment Google Scholar indexed journals CT articles CT Research articles CT review articles CT PubMed articles CT PubMed Central articles CT 2023 articles CT 2024 articles CT Scopus articles CT impact factor journals CT Scopus journals CT PubMed journals CT medical journals CT free journals CT best journals CT top journals CT free medical journals CT famous journals CT Google Scholar indexed journals Breast Cancer articles Breast Cancer Research articles Breast Cancer review articles Breast Cancer PubMed articles Breast Cancer PubMed Central articles Breast Cancer 2023 articles Breast Cancer 2024 articles Breast Cancer Scopus articles Breast Cancer impact factor journals Breast Cancer Scopus journals Breast Cancer PubMed journals Breast Cancer medical journals Breast Cancer free journals Breast Cancer best journals Breast Cancer top journals Breast Cancer free medical journals Breast Cancer famous journals Breast Cancer Google Scholar indexed journals haemorrhage articles haemorrhage Research articles haemorrhage review articles haemorrhage PubMed articles haemorrhage PubMed Central articles haemorrhage 2023 articles haemorrhage 2024 articles haemorrhage Scopus articles haemorrhage impact factor journals haemorrhage Scopus journals haemorrhage PubMed journals haemorrhage medical journals haemorrhage free journals haemorrhage best journals haemorrhage top journals haemorrhage free medical journals haemorrhage famous journals haemorrhage Google Scholar indexed journals Stem Cell Transplantation articles Stem Cell Transplantation Research articles Stem Cell Transplantation review articles Stem Cell Transplantation PubMed articles Stem Cell Transplantation PubMed Central articles Stem Cell Transplantation 2023 articles Stem Cell Transplantation 2024 articles Stem Cell Transplantation Scopus articles Stem Cell Transplantation impact factor journals Stem Cell Transplantation Scopus journals Stem Cell Transplantation PubMed journals Stem Cell Transplantation medical journals Stem Cell Transplantation free journals Stem Cell Transplantation best journals Stem Cell Transplantation top journals Stem Cell Transplantation free medical journals Stem Cell Transplantation famous journals Stem Cell Transplantation Google Scholar indexed journals
Article Details
1. Introduction
Floppiness is characterized by a reduced resistance to passive joint movement, and these infants clinically exhibit hypotonia in addition to motor developmental delays, hyper extensibility of joints, and abnormal postures [1]. Central hypotonia accounts for 60% of all cases of FIS, with the most common causes being perinatal asphyxia, infections, intracranial haemorrhage, metabolic disorders, and congenital cerebral malformations. Nonketotic hyperglycaemia (NKH), a defect in the glycine cleavage system, an enzyme complex found in the liver, brain, kidney, and placenta; it is also known as glycine encephalopathy [2]. 1:55,000 to 1:76,000 live births is the incidence rate [3]. The accumulation of glycine in all body tissues, particularly the cerebrospinal fluid. The majority of newborns appear normal at birth and are asymptomatic for a brief period. The infants show signs of rapid progression of neurological symptoms such as poor feeding, lethargy, seizures, and generalised hypotonicity [4]. Lissencephaly a gene-linked brain malformation, is characterised by the absence of normal cerebral cortex convolutions (folds) and an abnormally small head (microcephaly) in which cells fail to migrate to their appropriate destination. There were two types of lissencephaly: classic (Type 1) and cobblestone (Type 2). Classical Lissencephaly is uncommon, with an estimated prevalence of 12/ million births [5]. Other levels of dysfunction include anterior horn cells, neuromuscular junctions, peripheral nerves and muscles, which primarily leads to the development of peripheral hypotonia. Degeneration of the alpha motor neuron (α -motor neurons) in the anterior grey matter of the spinal cord causes Spinomuscular Atrophy (SMA), which manifests as progressive hypotonia and has a incidence of 1/10000 live births [6]. With a carrier frequency of 1 in 40–50, SMA is the second most common autosomal recessive disorder [7]. SMA patients have a homozygous loss of function of the survival motor neuron gene SMN1 on 5q13, but the modifying SMN2 gene remains functional. Because SMN2 produces fewer full-length transcripts, the number of SMN2 copies can affect the clinical phenotype, making it an important predictor of the type of SMA [8]. Neuromuscular junction disorders are broadly classified as presynaptic, synaptic, and postsynaptic [9]. Presynaptic is the rarest form (7-8%), while postsynaptic is the most common (75-80%) [10,11]. Agrin is a large proteoglycan, a nerve-derived factor that promotes the proper clustering of acetylcholine receptors in the neuromuscular junction. Clinical manifestations of agrin deficiency are similar to those of other postsynaptic forms of congenital myasthenic syndrome, such as ptosis, ophthalmoplegia, and proximal limb weakness. The diagnosis is confirmed by an electrophysiological study that shows decremented response, a morphological examination of muscle biopsy tissue, and testing for the ARGN gene mutation. There is no standard treatment protocol because of its rarity. Cholinesterase inhibitors and 3, 4-diaminopyridine have been found to be ineffective. Oral ephedrine at a dose of 2 mg/kg/day was found to be effective, but no studies have been conducted in India, as it is not easily available [11]. A variety of congenital myopathies (CM) affect the muscle's structural proteins, resulting in floppiness. There have been identified mutations in five genes coding for structural muscle proteins such as alpha-actinin, beta-tropomyosin, nebulin, troponin T1, and tropomyosin 2,3 [12]. Nemaline myopathies are characterised by non-progressive or slow-progressive muscle weakness and the formation of pathognomonic nemaline rods within muscle fibres (Autosomal dominant/recessive) presenting with hypotonia, proximal and facial muscle weakness, predominantly in the neck flexors [13]. The underlying cause of hypotonia is always challenging to determine for a clinician. Since there are many possible causes, approaching the case methodically and obtaining a thorough history and physical examination can aid in reaching a diagnosis. The determination of the aetiology is critical for management and prognosis. Only some of the causes of FIS have specific treatments, but in general, treatment consists of supportive care, such as rehabilitation, nutritional, and respiratory support.
2. Case Series
2.1 Case 1
Sixth born female baby to a 28 year old, second degree consanguineously married couple with a significant history of previous two sibling death and three abortions. Delivered at 38 weeks of gestation by caesarean section (CS) because of precious CS, with a birth weight of 2.6kg, the baby cried immediately after birth, and was shifted to Neonatal intensive care unit (NICU) in view of the refusal of feeds, lethargy, and hiccups. In NICU baby was received in a gasping state with bradycardia, hypothermia, and shock. CPR was started with bag and mask ventilation, and shock was treated as per protocol. On examination baby was hypotonic. Pupils were constricted, sluggishly reactive to light. Central nervous system (CNS) examination showed marked hypotonia of all four limbs with absent neonatal reflexes and deep tendon reflex. The sepsis screen was negative. Ammonia and lactate levels were normal. Arterial blood gas (ABG) showed mild metabolic acidosis, to rule out inborn errors of metabolism (IEM), workup was done. Tandem mass spectrometry (TMS) showed high levels of glycine of 2753.56 µmol/L in plasma. CSF analysis showed the normal picture, CSF glycine levels 423 µmol/L and CSF glycine: plasma glycine – 0.27 were high. Exome study showed homozygous variant c.1654A>G, in exon 13 of the GLDC gene. With these investigation findings and clinical features, we confined to the diagnosis of NKH (Non-Ketotic Hyperglycinemia), IEM. She was started on Sodium benzote and Dextromethorphan, along with cofactors, L carnitine, biotin, pyridoxine.
2.2 Case 2
A female born to a 19 year old primi mother, 3rd degree consanguinity, through CS in view of Grade II Meconium stained liquor (MSL). The baby weighed 1.9kg at birth and had a poor cry following tactile stimulation. In view of respiratory distress, the baby required minimal oxygen support through a nasal cannula for about 6 hours and then she was subsequently weaned to room air with normal saturation. The Sepsis screening was unremarkable. On physical examination, low-set ears and a prominent upper lip with a head circumference of 30 cm (< 3rd centile). On CNS examination, the tone of all four limbs were reduced, the baby had complete head lag when pulled to sit. The Moro, suck, palmar, and plantar reflexes were present with normal deep tendon reflexes. Further evaluation to find the cause for hypotonia, TORCH profile was done, which revealed an increased titre of IgG for cytomegalovirus (CMV)- 2.24 (Reference range- <0.8- Negative; 0.8 -1.2- equivocal; > 1.2- positive ) in the newborn.
MRI brain was performed, which showed:
- Brain surface smooth with gross loss of gyration and sulcation giving appearance of 8
- Foci of true diffusion restriction (hyper intense on DWI) in bilateral occipital lobe and left high parietal lobe.
- No gray matter thickening.

Figure 1: MRI brain: - Cerebrum shows shallow sylvian fissures and figure of eight appearance with smooth grey white interface and thickened cortex with virtual absence of sulcation.
This was consistent with Lissencephaly type 1 with hypotonia, and genetic test was adviced but could not be performed.
2.3 Case 3
A 13 day old girl , born to a 3rd degree consanguineous parents, delivered vaginally to 23 year old multigravida mother of P5L2D2A1, with a birth weight 2.40 kg, at 38 weeks and 5 days of gestational age came with jaundice and poor feeding since 2 days. The baby was admitted in NICU suspecting sepsis. Antenatal history uneventful. No history of NICU admission, but Mother gives history of weak cry and poor feeding since birth, since sucking efforts were poor, baby was fed with pallada. There was history of early sibling deaths and neurological disease in either parent’s family. Para 1 was medically terminated at one and half month, cause unknown. Death of two sibling (Para2 was a male and Para3 was female), in view of pneumonia at 1 year of age and both had global developmental delay with generalised hypotonia. Para4 is a male baby, doing well, presently 4 year old. Head to toe examination revealed low set ears and poor lip seal while sucking .On CNS examination, pith frog posture of the baby was observed. Baby had complete head lag when pulled to sit, and on vertical suspension there was a sensation of slipping through hands, with reduced tone of all four limbs including truncal hypotonia, and absent deep tendon reflexes. Mild tongue fasciculation was observed. Laboratory blood tests including complete blood count (CBC), lactate dehydrogenase (LDH), ammonia, creatine phosphokinase (CPK), thyroid, renal, and liver function tests were within normal limits. Genetic analysis revealed mutation of SMN gene, 3 copies of exon 7 and two copies of exon 8 identified in SMN2.Heterozygous duplication detected in exon 7 and no deletion /duplication detected exon 8 of SMN2 gene.
2.4 Case 4
A male term neonate weighing 3.2 kg was delivered by caesarean section due to non-progression of labour, to a 20-year-old primigravida, had a weak cry and no spontaneous effort at birth. The neonate received bag and mask ventilation for 1 minute and was intubated in view of poor respiratory efforts. The neonate was shifted to the NICU for further care and received mechanical ventilation. The baby was examined and found to be of appropriate gestational age, with equal and reactive pupils and a wide tongue. The tone was decreased in all 4 limbs with brisk reflexes. There were multiple episodes of convulsions on day 2 of life which were treated with phenobarbitone and levetiracetam as per the protocol. The convulsions were resolved on day 3 of life. The sensorium gradually improved and had spontaneous breathing efforts. A trail of extubation was given on day 4 of life, which failed. The baby also had multiple extubation failures from day 4 to day 68 of life, between tracheostomy was placed on day 49 of life. During these episodes, he had profuse sweating and temperature variability. Spinal cord injury was suspected and an MRI of the spine was done, which was normal. Blood investigations, including CBC, S.Electrolytes, S.Calcium, S.Magnesium and CPK were within normal limits. An ultrasound and a brain MRI were both normal. The 2D ECHO showed a small ventricular septal defect. A provisional diagnosis of congenital myasthenic syndrome was made. He was started on pyridostigmine, which his symptoms worsened and, hence, it was stopped. The baby was started on oral salbutamol with which he showed partial improvement. Exome sequencing showed it to be Autosomal recessive Congenital Myasthenic syndrome type 8 with a variant of uncertain significance in the AGRN gene, identified in the homozygous state P (Gly 1681 Arg) Exon 29 and additionally, likely pathogenic variant pCARE gene was identified in a homozygous state, with a genetic diagnosis of Autosomal Recessive Retinitis Pigments p.Gln 210 Exon 1. A diagnosis of autosomal recessive congenital myasthenia syndrome type 8 was made along with autosomal recessive retinitis pigmentosa.
2.5 Case 5
A male baby weighing 3kgs was born to a 26 year old, second degree consanguineous married couple with a significant antenatal history of polyhydramnios with decreased fetal movements noticed at 38 weeks. The baby was delivered by emergency CS (Indication – fetal distress-MSAF, with breech presentation). The baby did not cry immediately after birth and developed respiratory distress within the first three hours of life, necessitating intubation. Within 6 hours of birth, the baby began having seizures, which were treated with an AED and stabilized. On the sixth day of life, an attempt at extubation was made, but it failed. Subsequently, on day 10 and day 15 of life, extubation failure was noted. On general physical examination, the baby was alert and floppy with a frog-like posture and a lack of spontaneous movements noted, with myopathic facies-elongated face, wide open mouth, high arched palate, pectus excavatum, and contractures in both upper and lower limbs. In the CNS examination, the motor system examination revealed that the muscles of the facial expression were affected. Marked hypotonia of all four limbs and reduced reflexes. CPK was within Normal range, Muscle biopsy (Figure 2) was performed of the left rectus femoris, HE stained transversely cut skeletal muscle tissue showing polygonal to round fibers, peripherally placed nuclei and mild variation in fiber diameter; Modified Gomori Trichrome (MGT) stained transversely cut skeletal muscle fibers show polygonal to round fibers, peripherally placed nuclei, mild variation in fiber diameter and subsarcolemmal red stained nemaline rod bodies; electron microscopy showed Transversely cut skeletal muscle fibers. Stained with Uranyl acetate & lead citrate showing several electron dense rod bodies with the final report suggestive of Nemaline rod myopathy.
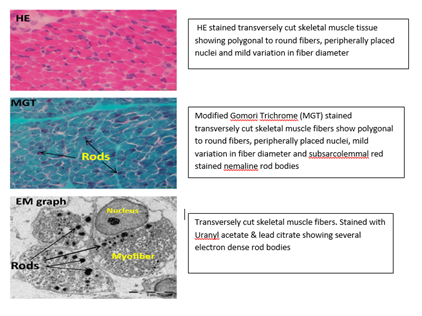
Figure 2: Neuro-histopathology of the Muscle Biopsy.
3. Discussion
Floppy infant syndrome, also known as rag-doll syndrome, is characterised by hypotonia secondary to peripheral or central nervous system involvement. We herein discuss the case study of five distinctive presentations of FIS that were diagnosed to have Non–ketotic hyperglycinemia, lissencephaly, spinomuscular atrophy, congenital myasthenia gravis, and nemaline rod myopathy with involvement of the brain (IEM and Structural), spinal motor neuron, neuromuscular junction, and muscle, respectively. Non-ketotic acidemia is a rare disease that has been reported to be more common in Northern Finland, British Columbia, and Israel [14]. Patients with NKH presents with lethargy, hypotonia, and poor sucking, which can progress to apnea, hiccups, myoclonic seizures, and the need for assisted ventilation. The majority of NKH patients die during the neonatal period [15]. Survivors develop seizures that are uncontrollable and mental retardation. In the absence of an organic acid disorder, hyperglycinemia and elevated CSF glycine levels, diagnose this condition. Since there is no absolute cure, and the treatment targeted at decreasing glycine burden include protein restriction, a synthetic diet devoid of glycine, and benzoate, strychnine, and benzodiazepines to promote renal excretion, as well as antagonism of glycine's neurotransmitter effect. Valproate, among the AEDs, should be avoided in patients with NKH because it raises blood and CSF glycine concentrations by inhibiting the GCS further and may increase seizure frequency [16]. Our case had no seizures, but presented classically with hiccups, refusal of feeds and lethargy and elevated CSF glycine levels. Minimal cases have been reported in India as there is paucity of data due to early neonatal death and lack of work up in resource limited settings. The cortex thickens in classical lissencephaly (or type I), with four more or less disorganised layers rather than six normal layers; however, if they are infected with TORCH, the cortex appears thin. Extra-cortical anomalies (total or subtotal agenesis of the corpus callosum and/or cerebellar hypoplasia) are also present in classical lissencephaly variants. On the basis of their genetic aetiology, four types of lissencephaly can be distinguished: LIS1 gene aberrations (isolated lissencephaly and Miller-Dieker syndrome), TUBA3 and DCX gene aberrations, and ARX gene mutations (XLAG syndrome, X-linked lissencephaly with agenesis of the corpus callosum) [17]. Non-genetic causes include TORCH infections, among which CMV constitute 10%. Our case is classical lissencephaly with a prominent upper lip and hypotonia. Seizures occur in over 90% of Lissencephaly with onset before 6 months in about 75% of the cases [18] and more than half of these present with infantile spasms. Interestingly, the baby had no seizure episodes. Generally, other neurological manifestations include profound mental retardation and hypotonia that evolves to spasticity with time which requires regular follow-up and developmental assessment. Spinal muscular atrophy (SMA) is an autosomal recessive disorder that causes progressive proximal muscle weakness and paralysis. It is caused by the degeneration of alpha motor neurons in the spinal cord [19]. In our case, SMN2 duplication was discovered in exon 7, but SMN1 was not involved. Generally, SMN2 duplication is a milder form of SMA. In contrast, our case manifested as hypotonia in the neonatal period. This suggests that even a minor form of SMA can cause hypotonia and feeding difficulties. Development, systemic complications due to muscle weakness associated with SMN2 duplication shall be assessed during follow up period. Congenital myasthenic syndromes (CMSs) are currently caused by mutations in 32 genes that are autosomal dominant or autosomal recessive. These mutations affect eight presynaptic proteins, four synaptic proteins, fifteen post-synaptic proteins, and five glycosilation proteins. Ion channels, enzymes, structural, signalling, sensor, and transporter proteins are all examples of these proteins. CHAT, COLQ, RAPSN, CHRNE, DOK7, and GFPT1 are the most common causative genes. These mutations cause abnormal fatigability, hypotonia, or developmental delay, as well as permanent or unstable weakness of extra-ocular, facial, bulbar, axial, respiratory, or limb muscles [20]. Only three reports exist that describe a congenital myasthenic syndrome caused by argin defects. One study found that two siblings with a homozygous missense mutation (G1709R) in the laminin G-2 domain, which is required for MuSK activation, had ptosis and mild weakness of the facial and limb girdle muscles. Pyridostigmine and 3,4-diaminopyridine did not work. Second, two heteroallelic mutations in the N-terminal region of Q353X and V1727 in the laminin G domain of argin caused severe congenital myasthenic syndrome. Pyidostigmine and 3,4-diaminopyridine were also ineffective in this patient, but ephedrine had a partial effect [21]. Another study found that pathogenic variants in the AGRN gene cause 1% of congenital myasthenic syndrome, which was reported in 7 families [22]. In our case, congenital myasthenic syndrome presented with clinical manifestations of a wide-open mouth, ptosis, muscle weakness of all four limbs, hypotonia, with brisk reflexes and multiple extubation failures, gene analysis showing the rarest form of congenital myasthenic syndrome with ARGN gene mutation in homozygous state p(Gly 1681 Arg) Exon 29, being autosomal recessive type 8 with a variant of uncertain significance, along with pCARE gene identified in the homozygous state with autosomal recessive retinitis pigments. p. (Gln 210*) Exon 1, being the rarest combination of the congenital myasthenic syndrome and retinitis pigmentosa. Despite the fact that ephedrine is an effective drug, we started the baby on oral salbutamol as ephedrine was unavailable, and the baby improved clinically at first but response phased off over time. Nemaline myopathies (NM) can manifest at various stages of life. Severe congenital form occurs at birth with severe hypotonia, muscle weakness, feeding difficulties, and may be associated with dilated cardiomyopathy and arthrogryposis; the majority of these babies die in utero. Intermediate idiopathic forms fail to achieve motor milestones, do not develop joint contractures, and require respiratory support by the age of 11, making them wheelchair bound. Only four cases of NM were identified in a report from the National Institute of Mental Health and Neurosciences (NIMHANS), Bangalore, South India, which reported 100 cases of congenital myopathies diagnosed over a 20-year period (with a total of 6241 muscle biopsies examined) [23], in contrast to a report from AIIMS, North India, which identified four NM cases (26.6 percent) out of 15 CM cases (out of the 750 muscle biopsies performed over a 18-month period) [24]. ACTA1, the gene for alpha-actin, is thought to be involved in 60 to 75 percent of cases of severe congenital nemaline myopathy. The majority of the remaining cases are caused by nebulin defects, are autosomal recessive, and manifest as typical congenital NM [20]. Mutations in the ACTA1 gene are linked to a variety of clinical, pathological, and genetic findings. [25,26] As a result, this case report emphasises the clinical, histochemistry, and ultrastructural examination for the diagnosis of Nemaline Myopathy, a rare congenital myopathy.
4. Conclusion
Through this case series, we want to provide insight into the complicated nature of floppy syndrome in neonates and an understanding of any therapeutic possibilities that might be available. We made an effort to connect a statement- “A chain is no stronger than the weakest link”, to our case series; which included examples with the involvement of the brain, spinal motor neuron, NMJ and muscle; all ultimately resulted in hypotonia in neonates. Paediatricians and Neonatologists must recognise hypotonia early in the neonatal period and search for the underlying causal syndrome to provide effective treatment.
Ethics Approval and Consent to Participate
This is a case series were patient approval was taken.
Consent for Publication
The authors consent is present for publishing this case series.
Competing Interests
There is no conflict of interest.
Funding
No funding.
Authors' Contributions
Dr.Surabhi: Editing and Reviewing, Dr Adarsh E: Editing and reviewing, Dr.Sagethya. : Writing original draft, editing, Dr Prathik BH, Dr Niranjan HS and Dr Naveen Benakappa:Helping in making this case series.
Acknowledgements
I would like to express our gratitude to Adarsh E, Professor and HOD Department Of Pediatrics and other contributers who helped me recruiting participants and collecting data for the study.
References
- Floppy infant syndrome.Igarashi MJ Clin Neuromuscul Dis 6 (2004): 69-90.
- Hamosh A, Johnston M. Nonketotic hyperglycinemia. In: Scriver CR, Beaudet A, Sly W, Valle D, editors. The Metabolic & Molecular Bases of Inherited Disease. 8th ed.. New York: McGraw-Hill (2001): 2065-2078.
- Coughlin CR 2nd, Swanson MA, Kronquist K, et al. The genetic basis of classic nonketotic hyperglycinemia due to mutations in GLDC and AMT. Genet Med 19 (2017): 104-111.
- Tada K, Narisawa K, Yoshida T, et al. Hyperglycinemia: A defect in glycine cleavage reaction. Tohoku J Exp Med 98 (1969): 289-296.
- Parrini E, Conti V, Dobyns W, et al. Genetic Basis of Brain Malformations. Mol Syndromol 7 (2016): 220-233.
- Groen EJN, Talbot K, Gillingwater TH. Advances in therapy for spinal muscular atrophy: promises and challenges. Nat Rev Neurol 14 (2018): 214-224.
- Keinath MC, Prior DE, Prior TW. Spinal Muscular Atrophy: Mutations, Testing, and Clinical Relevance. Appl Clin Genet 14 (2018): 11-25.
- Omar A, Marwaha K, Bollu PC. Physiology, Neuromuscular Junction (2022).
- Engel AG. Congenital myasthenic syndromes in 2012. Curr Neurol Neurosci Rep 12 (2012): 92e101.
- Barisic N, Chaouch A, Muller JS, et al. Genetic heterogeneity and pathophysiological mechanisms in congenital myasthenic syndromes. Eur J Paediatr Neurol 15 (2011): 189e96.
- Nance JR, Dowling JJ, Gibbs EM, et al. Congenital myopathies: an update. Curr Neurol Neurosci Rep 12 (2012):165-174.
- Sewry CA, Laitila JM, Wallgren-Pettersson C. Nemaline myopathies: a current view. J Muscle Res Cell Motil 40 (2019): 111-126.
- James J. Dowling, Alan H. Beggs, in Neuromuscular Disorders of Infancy, Childhood, and Adolescence (Second Edition) (2015).
- Applegarth D, Toone J. Non Ketotic Hyperglycinemia ( Glycine Encephalopathy ): Laboratory Diagnosis, Molecular Genetics and Metabolism 74 (2001): 139-146.
- Tastekik A, Rahmi Ors, Ozkaa B, et al. A Rare Case of Neonatal Hypotonia: Neonatal Non Ketotic Hyperglycinemia. Turk J Med 32 (2002): 73-75.
- Morrison PF, Sankar R, Shields WD. Valproate-induced chorea and encephalopathy in atypical nonketotic hyperglycinemia. Pediatr Neurol 35 (2006): 356-358.
- Verloes A, Elmaleh M, Gonzales M, et al. Lissencéphalies: aspects cliniques et génétiques [Genetic and clinical aspects of lissencephaly]. Rev Neurol (Paris) 163 (2007): 533-547.
- Guerrini R, Filippi T. Neuronal migration disorders, genetics, and epileptogenesis. J Child Neurol 20 (2005): 287-299.
- D'Amico A, Mercuri E, Tiziano FD, et al. Spinal muscular atrophy. Orphanet J Rare Dis 6 (2011): 71.
- Finsterer J. Congenital myasthenic syndromes. Orphanet J Rare Dis 14 (2019): 57.
- Engel AG, Shen X, et al. Congenital Myasthenia Syndromes:Pathogenesis, diagnosis, and treatment. Lancet Neural 14 (2015): 420-434.
- Huzé C, Bauché S, Richard P, et al. Identification of an agrin mutation that causes congenital myasthenia and affects synapse function. Am J Hum Genet 85 (2009): 155-167.
- Nemaline rod myopathy: A rare form of myopathy (2007).
- Ryan MM, Schnell C, Strickland CD, et al. Nemaline myopathy: A clinical study of 143 cases. Ann Neurol 50 (2001): 312-320.
- Agarwal PB, Strickland CD, Midgett C, et al. Heterogeneity of nemaline myopathy cases with skeletal muscle alpha-actin gene mutations. Ann Neurol 56 (2004): 86-96.
- Marston S, Mirza M, Abdulrazzak H, et al. Functional characterization of a mutant actin(Met132Val) from a patient with nemaline myopathy. Neuromuscul Disord 14 (2004): 167-174.